On July 26, Shoukhrat Mitalipov woke up to headlines about his research: “First human embryos edited in US,” said one; “Genome of viable human embryos edited in controversial study,” said another. Normally, this would be a cause for celebration for the Oregon Health & Science University (OHSU) professor. But in this case, all the media coverage did was stress him out—his study wasn’t supposed to be published until Aug. 2.
“I don’t know how it got leaked,” Mitalipov said on July 28. “It’s likely because of a combination of hot words: Crispr, gene-editing, and designer babies.”
He sounded annoyed, and justifiably so. The articles had rung alarm bells about “designer babies” engineered to have advantageous genetic traits, but his research was far from proving that a possibility. Although it did involve the use of human embryos—which is a union of egg and sperm—it also wasn’t particularly controversial. In 2015, after a long debate, experts at the US National Academies of Science, Engineering, and Medicine concluded that this sort of research on human embryos should be encouraged. And in fact, one prominent news story that called Mitalipov’s study “controversial” actually contained testimonies from two bioethicists who supported the study (the headline has since been changed).
Today (Aug. 2), Mitalipov’s study was published in the journal Nature. It shows the first highly successful use of the gene-editing technique called Crispr to improve human health: his team was able correct a genetic mutation that causes a life-threatening cardiac disease. None of the embryos were allowed to come to term. But if Mitalipov has his way, future projects could eradicate a disease that affects millions—one in 500 people carry the mutation—and even kills unsuspecting, seemingly fit adults.

Picking the target
On Mar. 4, 1990, Loyola Marymount University basketball player Hank Gathers dunked the ball to put the Loyola Lions well ahead of the University of Portland Pilots. Immediately afterwards he collapsed and stopped breathing. There were 13 minutes left in the basketball game; he’d given everything to the sport. The cause of death was later determined to be hypertrophic cardiomyopathy (HCM), the most common inherited cardiac disease and the most common cause of death in young athletes.
Mitalipov began working on the project three years ago. He knew he wanted to try to use Crispr to cure a genetic disease, but first he needed to pick which mutation in the human genome to target. He could have chosen from thousands, but Sanjeev Kaul, a cardiovascular specialist at OHSU, convinced Mitalipov to go after the HCM gene mutation.
“It’s such a terrible disease to deal with it, and it’s present in a large population,” Mitalipov says. “In India, for instance, nearly one in ten people carry it.”
The most common cause of HCM is a genetic mutation in one of nine genes. Mitalipov’s research shows that you can take a human embryo with one such mutation, where the human genome is missing four base pairs (or letters of DNA) and simply correct it. In theory, if you let that embryo grow, it would develop into a human without HCM.
Substitutes won’t do
Next Mitalipov had to convince the OHSU review board to approve the use of human embryos. That part took about two years.
Most research to understand what happens at the embryonic stage of human development is done on embryo-like cells, created by exposing adult stem cells to a special handful of chemicals. But though embryo-like cells have been a boon for research, they are not a perfect replacement for embryonic cells. Certain things, like DNA repair systems, can be quite different in embryos than they are in embryo-like cells.
And DNA repair was key to Mitalipov’s research. Crispr is the most precise gene-editing technique we have, but it doesn’t actually “edit” genes. Instead, it seeks out the gene it has been programmed to target and then makes a snip. The next step almost always involves relying on the cell’s own DNA repair system, which finds the cut and does its best to fix it.
In most cells in the body, there are two repair systems. The system most often used by the body requires less effort, but it also does a sloppy job: sometimes inserting a letter of DNA where it doesn’t belong and other times deleting a letter of DNA that should be there. The other repair system produces more accurate results, but the cells need to put in more effort to make it happen, so it’s rarely used. However, past research hinted that human egg cells tend to use the second system. Mitalipov needed this especially strong DNA repair system to accomplish his Crispr-HCM edit.
With the case made and approval secured, Mitalipov’s team went into full gear. A group of collaborators recruited a man with the HCM mutation who would donate sperm, and a dozen healthy women who would donate eggs for the research.

Beating China
Three previous studies that used Crispr in human embryos—all conducted in China—produced poor results. The first, published in 2015, injected Crispr in 86 non-viable embryos (all discards from in-vitro fertilization treatment), in an effort to cure the inherited blood disorder β-thalassemia. It only worked in about 33% of the samples, and even then in those cases it was far from perfect: each had undesirable cuts in other locations on the genome (called “off-target effects”).
Another attempt published in 2016 tried a different tack. Instead of removing a mutation, it sought to induce one that would provide resistance to HIV. Gene insertion was only successful in 25% of the 26 non-viable embryos, and many of those “successes” ended up with the wrong mutation.
Earlier this year, the researchers made the case that the reason their 2016 study failed wasn’t because Crispr wasn’t doing its job. Instead, they suspected that the embryos used, because they were non-viable, were too abnormal to effectively test Crispr’s precision. But in a new study using six viable human embryos, they were still able to fully correct the mutation in only one. In another two, they fixed the mutation but in only some of the cells of the embryo—leading to a condition known as “mosaicism.” In these cases, if the embryo were to come to term, it would create a human whose body has some cells with the mutation and others without. The upshot would be that there’s no guarantee the adult would be free of the disease.
Mitalipov had a plan to avoid both off-target effects and mosaicism. Instead of injecting Crispr after the embryo had formed, he decided to make the snip during the process of fertilization. So while injecting sperm, he also injected Crispr.
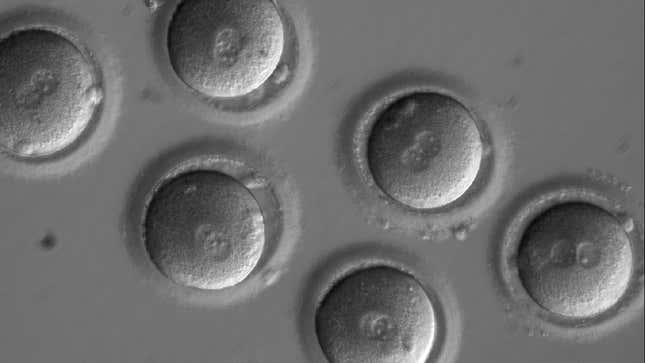
The programmed Crispr system located the position in the sperm DNA where the man’s genome was missing the four key base pairs and it made a simple cut there. The fertilization process began with the cut in place. However, at some point during the early stages of the development of the embryo—and Mitalipov doesn’t know exactly when—the egg’s DNA repair system kicked in. The new embryo contained one strand of DNA from the mother, which the team knew to be healthy and without the HCM mutation, and another strand from the father that included a snip at the location of the HCM mutation.
When the egg’s more accurate DNA repair system detects the cut in the paternal strand, it started fixing it, using the maternal strand as a model. And because the maternal strand was healthy, the repair system added the four missing base pairs to the paternal strand, and the HCM mutation in the embryo was corrected. (This method is only applicable to situations where one parent has the mutation; if both parents have the same mutation, this type of DNA repair system has no functional model to copy.)
Mitalipov’s team tested their technique on a total of 163 viable embryos, and the mutation was corrected successfully in nearly 50% of them. Better still, only one of those embryos showed mosaicism and none showed any off-target effects.
Two independent experts Quartz contacted—Dieter Egli of Columbia University and Maria Jasin of the Memorial Sloan Kettering Cancer Center—weren’t sure Mitalipov’s claims were water tight. Based on the information provided in the study, Egli couldn’t confidently say for sure that the tests used to detect mosaicism caught all mosaics. In other words, it’s possible that results appear to be more positive than they actually are. Jasin pointed out that more evidence was needed to back up the claim that it was the more accurate method of DNA repair that the embryo used. She acknowledged that working with human embryos has extra constraints because researchers need to justify the use of every extra embryo, but still felt there were some gaps in the study.

The father of human genetic engineering
If the results do stand up, they are orders of magnitude better than the three previous Chinese attempts—both because of the rate of success and the number of viable embryos tested. But Mitalipov acknowledges that it’s still not good enough. “A 50% success rate is too low,” he says.
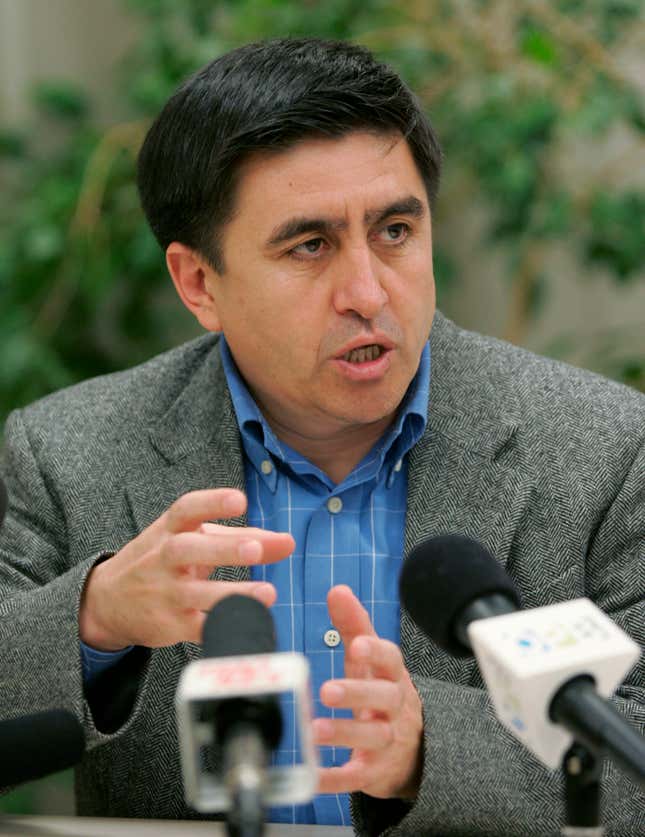
His exacting standards have paid off in the past. Mitalipov’s groundbreaking work in the late 2000s paved the way for “three-parent babies;” last year, a technique he developed contributed to the birth of the first genetically engineered baby born with DNA from two females and one male.
The technique cures mitochondrial disease, which is caused because of faulty mitochondria, the power packs in each human cell that converts sugar into a usable form of energy. Children born with mitochondrial disease either die young or live disabled lives.
Most human genes are jammed into the cell’s nucleus, but there’s a tiny fraction (37 out of 20,000) present in mitochondria. These genes are only passed down from mother to child. Mitalipov developed the technique to replace a mother’s faulty mitochondria with healthy ones from a female donor. Though the baby will mostly still be the genetic child of one man and one woman, he or she will have a small number of genes from another woman, technically a third “parent.”
“In 2009, when I published my research on mitochondrial replacement, people laughed at me,” he says. “They said ‘You will never bring it to clinic.’ Look where we are today.”
He first developed the technique in monkeys, and then progressed to human embryos—reaching success rates of 99%. He has the same ambition for Crispr-edited human embryos.
“I have a very strong opinion on clinical applications. I didn’t do this research to satisfy my curiosity,” Mitalipov says. “This was done to develop the technology and bring it to clinics. It may take a decade, but we will be there.”

Slippery slope?
Crispr-editing of human embryos is a much more complex endeavor than the three-parent baby technique. There is no guarantee, for instance, that the steps used by Mitalipov to fix the HCM mutation would work on other similar mutations or on more complex ones. However, if the technique is perfected, it will have a much greater impact on human health than anything the three-parent technique could ever offer.
The complexity is also a safeguard against the use of the technique to, say, create babies with traits such as higher intelligence or greater strength. We don’t yet understand the sheer number of genes involved in the creation of those traits, let alone what it would take to make such detailed edits to the human genome. In other words, even if you think it’s an ethical hazard to the Crispr to provide advantageous mutations, there’s no reason to worry right now; those “designer babies” are decades away.
A more immediate threat is unregulated use. Genetic engineering of humans has already begun, and there is real danger in people with inadequate understanding of the risks starting to use Crispr to edit out inherited illnesses and bring the embryos to term. The baby is likely to suffer mosaicism, off-target effects, or other as-yet-undetected consequences, and we don’t know what that could do to the person born with them.
The first three-parent baby was born in Mexico, because at the time the country had no regulations on the use of the technique. Though the baby was reported to be healthy few months after birth, the risks of things going awry are much higher without the rigorous checks provided by clinical trials in a country with strong regulations. (The second three-parent birth was reported to have occurred in Ukraine.)
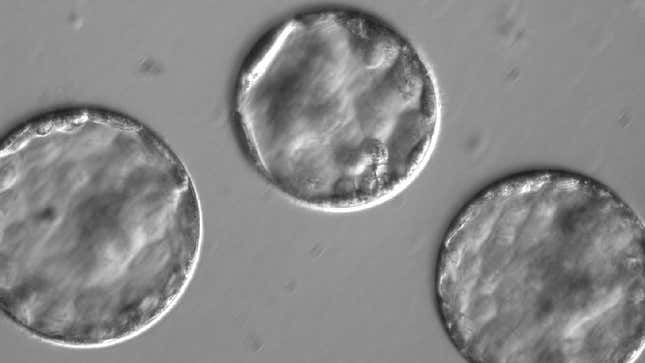
“Unfortunately, that’s a risk,” admits Paula Amato, a gynecologist who collaborates with Mitalipov at the OHSU. “Obviously, we would prefer clinical applications occur in a regulated environment. But it’s not something we can control.” In the US, the Food and Drug Administration (FDA) forbids the use of human embryos in clinical trials. “So it’s not surprising to me that people would attempt to circumvent such restrictions,” Amato says.
In the US, only pre-clinical research involving human embryos is allowed and even for that government funds aren’t available. Mitalipov’s work was supported mostly by OHSU’s institutional funds and the rest through charitable foundations. His collaborators in South Korea, where human embryo research is strictly forbidden, had to get lawyers and were only able to handle DNA extracts from the test embryos, and not the embryos themselves.
There are some places that offer regulated environments without stifling experimentation and clinical use. Amato points to the UK, the first country to offer licenses to create three-parent babies, if those applying could make the case for it. The country spent years debating the topic among experts and holding public consultations before it reached that stage.
Ultimately, if society would like to see the use of Crispr or other techniques to rid humanity of devastating diseases, countries will need to undertake a process similar to what the UK went through. Without hearing the voice of the public and putting the right regulations in place, the fear of “designer babies” could not only lead to dangerous experimentation in unregulated environments, but also quash the true potential of this powerful tool.