Everyone has seen the periodic table. The colorful list of all the elements in the universe, found in the back of chemistry textbooks and on nerdy posters, is immediately recognizable to anyone who has gone through high school. It may surprise you, however, to learn we’ve had no proof where most of the elements on the table come from.
We can find almost all of these elements on Earth, yet we don’t know how our little planet got them. They certainly didn’t form here. Almost everything on the Earth is borrowed either from dust left behind during the formation of the solar system or received in the form of comets and asteroids crashing into the planet. (The only elements that get made on Earth are those that don’t occur naturally, are created by humans in large scientific laboratories, and exist for fractions of a second.)
Where could they come from?
To forge new stable elements, you need extreme amounts of energy that will crush and put the sub-atomic components of elements—protons, electrons, and neutrons—together in new configurations. (Remember, an element differs from another merely because it has a different number of protons.)
So the next obvious candidate would be our neighborhood star. The sun is large and exacts tremendous pressure. Might it produce all of the elements in the periodic table? Nope, our sun is too small for the kinds of energy needed to produce heavier elements. The only elements you can find on the sun are hydrogen, helium, lithium, beryllium, and boron. We have to look elsewhere for the origins of the remaining 89 elements that naturally occur on Earth.
Are the sources something larger? Yes and no. We know that stars that are at least 10 times the mass of our sun are able to make some more elements and some are made in exploding stars. These include those with atomic numbers between carbon and zirconium. That still leaves 54 other elements.
Scientists have theorized that these remaining elements are made in even more violent celestial events—and now they have proof. Today (Oct. 16), in a series of papers published in many landmark journals, hundreds of scientists tell the story of observing one of the most epic events in the universe: the violent merger of two neutron stars.
The drama of a neutron-star merger is due to the fact that it involves one of the most extreme objects in the universe. Neutron stars are some of the smallest, densest stars we know. They do not have much more mass than our sun, but all of it is compressed into a ball no bigger than the width of a mid-sized city (about 15 km, or 9 miles). That’s a lot of compression. A teaspoon of neutron star would weigh 10 billion kg (or 22 billion lbs)—about the same as 1 million very large elephants.
When two of these objects happen to come close enough to one another, their super-strong gravities tug on each other, creating disturbances in space-time called gravitational waves.
What Einstein envisioned
Think of it like this: As Albert Einstein first posited, the universe can be conceived of being made of a fabric of space and time. Just as a fabric made of cotton would bend if you pulled it taut and then put a heavy ball on it, space-time bends around very heavy objects. When two such heavy balls interact with each other, they create ripples in space-time that travel outwards into the universe.
On Aug. 17 this year, scientists at LIGO (the Laser Interferometer Gravitational-wave Observatory) saw, for the first time, signs that this sort of event was happening. Here’s how they detect those signs, as Quartz explained previously:
Each detector consists of two 4-km long tunnels at right angles. Each tunnel is emptied of all air. A laser beam is split in two, and each beam is sent down one tunnel and reflected back, where they are recombined. Gravitational waves stretch and then contract spacetime… As they passed through the LIGO team’s lasers, they changed, ever so slightly, the times it took each laser to travel those four kilometers. The distortion would only be as wide as a fraction of the width of a single atom. But that was enough to change the pattern of the laser light where the two beams were recombined.
Using these tiny deviations, scientists were able to identify the event as the merger of two neutron stars about 130 million light years away—that is, it occurred 130 million years ago, and because gravitational waves move at the speed of light, they only just reached the Earth.
The four previous times LIGO scientists detected gravitational waves they belonged to the merger of black holes, which are the only objects in the universe denser than neutron stars. LIGO scientists were expecting to see neutron stars merge, and were ready to differentiate it from other events that can produce gravitational waves, such as supernovae (exploding stars). The timing of this major announcement is serendipitous: The scientists who built LIGO just won this year’s Nobel Prize in Physics.
The serendipity of a neighborhood event
The LIGO scientists observed these waves for about 100 seconds—before the moment the two neutron stars crashed into each other. Two seconds after that, gamma rays from the stellar merger reached the Earth. Over the next days and weeks, the event was recorded by 70 ground- and space-based observatories that LIGO had alerted. That’s special, because blackhole mergers don’t release visible light. That’s why this data from a merger of a neutron star would provide LIGO scientists information they had never seen before.
“On the cosmological scale, this happened in our neighborhood,” says Andreas Freise of the University of Birmingham and a LIGO collaborator. It’s good luck, then, also on a cosmological scale that humans happen to have invested in building the right instruments to watch such an event at just about the right time. (The instrument had been expecting such a neutron-star merger, but researchers had thought it would likely have occurred much farther away.)
In the days to follow, other types of electromagnetic radiation—including X-ray, ultraviolet, optical, infrared, and radio waves—were detected. Each element has a unique electromagnetic signature. Scientists were able to detect signatures related to an occurrence called the “r process” which was thought to create heavy elements. It’s here that elements like gold, platinum, and uranium are made.
“This detection has genuinely opened the doors to a new way of doing astrophysics,” Laura Cadonati, professor of physics at Georgia Tech and LIGO collaborator, said in a statement. “I expect it will be remembered as one of the most studied astrophysical events in history.” And now you, too, know where in the universe all the elements of the periodic table get made.
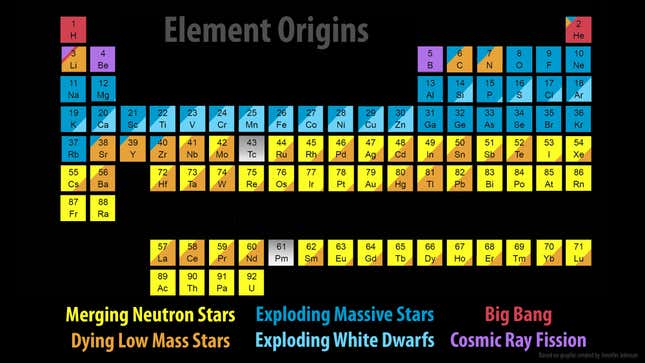