Is the universe pro-life?
An old and profound existential question is receiving new interest from the scientific community: Was the emergence of life in the universe an improbable event, or the opposite, an inevitable one? In other words, did life occur as a result of chance and contingency, or was it an inescapable and predictable consequence of natural law?
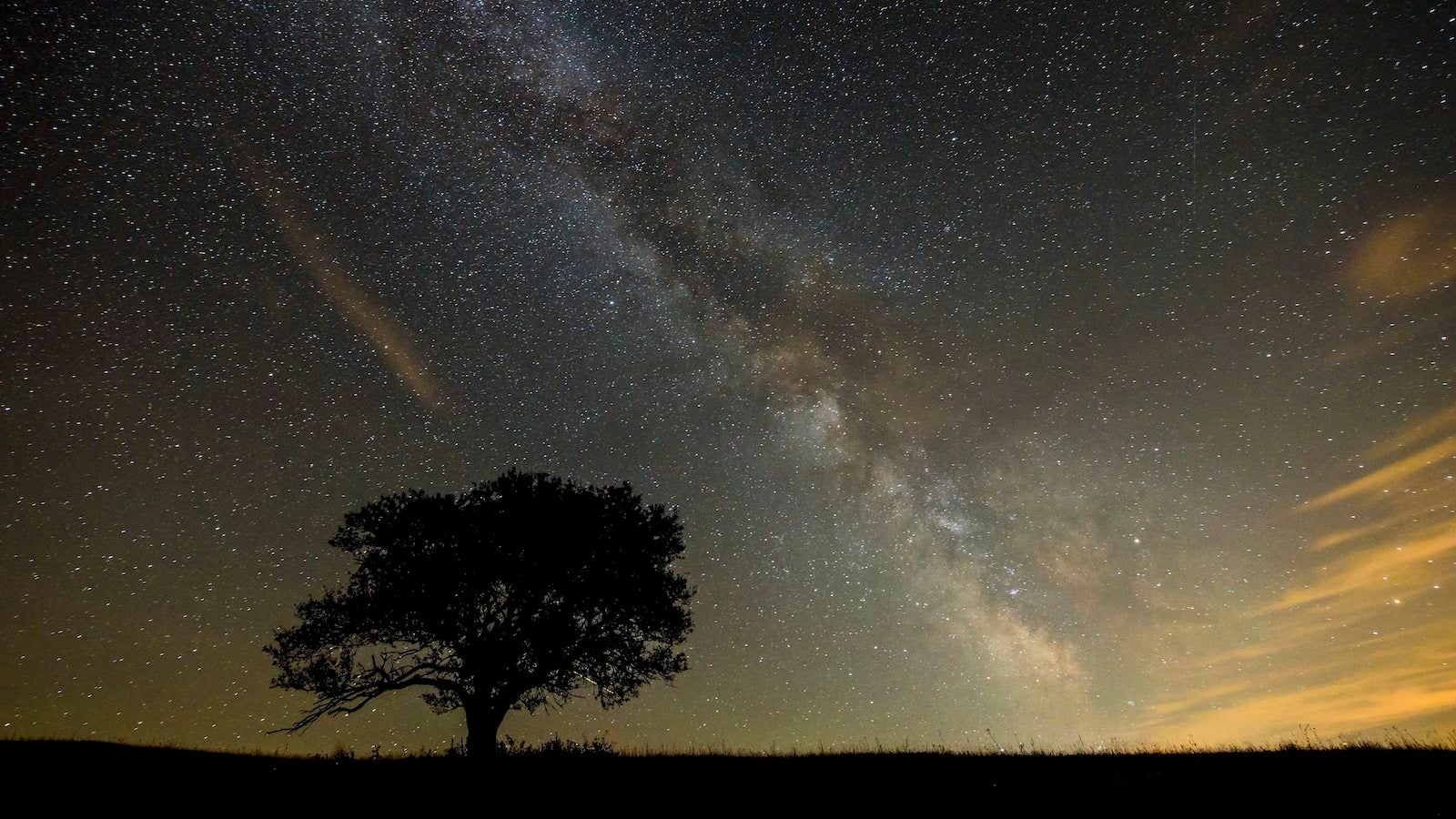
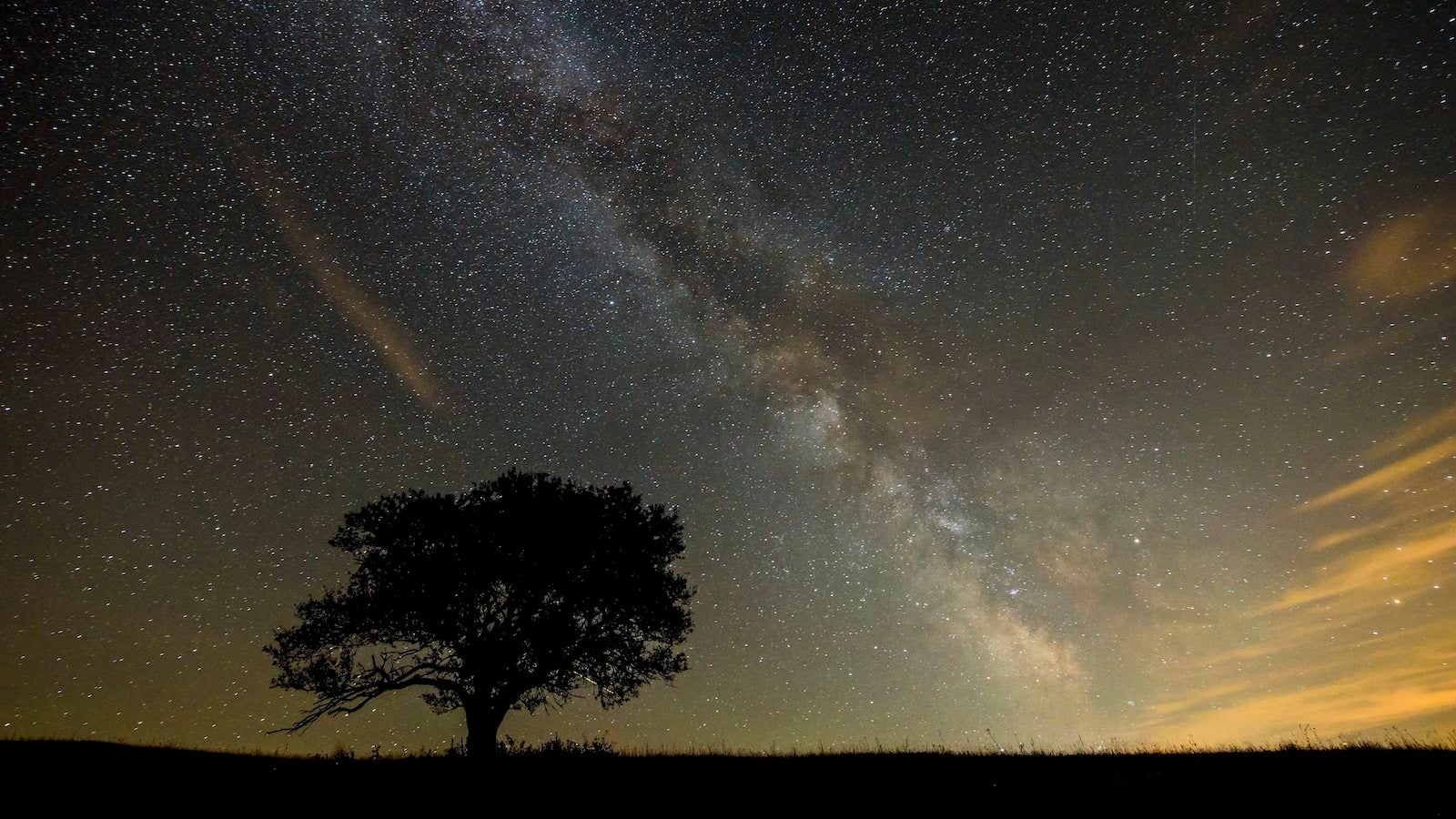
An old and profound existential question is receiving new interest from the scientific community: Was the emergence of life in the universe an improbable event, or the opposite, an inevitable one? In other words, did life occur as a result of chance and contingency, or was it an inescapable and predictable consequence of natural law?
The answer to this question would tell us whether biological life is a fluke or a regularity. The former answer would suggest that we are alone in the universe, a statistical anomaly. The latter suggests that the phenomenon is not uncommon in the cosmos, likely occurring on other planets with sufficiently Earth-like conditions.
While this question might first appear impossible to answer due to a severely limited sample size—Earth is the only life-harboring planet we know of—a new understanding of the mechanisms underlying the origin of life is reinvigorating the notion of a bio-friendly universe.
But before getting into those mechanisms, some historical context may help put the question into perspective.
The improbable vs. inevitable debate
The mainstream scientific worldview assumes that life on Earth was an unlikely event that occurred against all odds. This “frozen accident,” which resulted from an improbable molecular collision in a primordial soup, likely wouldn’t happen again if we could rewind the tape of the universe and replay it with even the slightest change.
This assumption rests on the idea that Darwinian evolution is the exclusive means of physical adaptation in nature; that all the diversity and complexity we see in and around us, broadly construed, can be explained by environmental pressures acting on random genetic mutation. According to this paradigm, since adaptive change requires genes, the emergence of life itself must have been a result of chance, rather than an evolutionary process.
The Nobel prize-winning French biologist Jacques Monod—a staunch skeptic and atheist—held the passionate and uncompromising belief that “The universe was not pregnant with life.” He poetically summed up this worldview in his influential book Chance and Necessity, published in 1970:
“The ancient covenant is in pieces; man knows at last that he is alone in the universe’s unfeeling immensity, out of which he emerged only by chance. His destiny is nowhere spelled out, nor is his duty.”
But there is an alternative worldview gaining traction that is equally scientific and perhaps better supported by empirical evidence: If the physical and chemical conditions on Earth some 4 billion years ago were ripe for biological life, then it would serve to show that life in the universe is not a cosmic accident, but a cosmic imperative.
The “inevitable life” school of thought is based on the idea that there are thermodynamic factors that constrain the random motion of atoms and molecules in such a way that essentially guarantees that biological systems will emerge where conditions permit. As an “attractor”—a state toward which a dynamical system tends to evolve—one could say that life was written into the “cosmic code.”
This view was first articulated by another Nobel prize-winning French biologist from the 20th century, Christian de Duve. In his exceptionally cogent book Vital Dust, he argued that Monod’s claim that the universe wasn’t pregnant with life was not just wrong, but provably wrong.
Decades after his book was published, physicists studying the origin of life from the Santa Fe Institute and MIT are arguing that de Duve’s position should be the reigning one. These researchers contend that the emergence of biological systems was an event that could have been predicted, and their reasoning is based on the fundamental physics principle known as the second law of thermodynamics.
The second law states that the amount of entropy in the universe increases over time. But there is quite a bit of confusion over exactly what is meant by the term “entropy,” as there are numerous definitions used in different contexts. As it applies to the universe, the second law merely states that the finite amount of energy in the universe naturally tends to spread out. In doing so, the amount of “free energy” in the universe, meaning the energy that is available to do physical work, inevitably decreases over time. Entropy is simply a measure of the amount of energy no longer available to do work.
It’s a new understanding of this old and fundamental law of physics, particularly in how it relates to the emergence of complexity, that is changing how we think about the probability of life in the universe.
Dissipative adaptation: How energy flows create order
According to the inevitable life theory, biological systems spontaneously emerge because they more efficiently disperse, or “dissipate” energy, thereby increasing the entropy of the surroundings. In other words, life is thermodynamically favorable.
As a consequence of this fact, something that seems almost magical happens, but there is nothing supernatural about it. When an inanimate system of particles, like a group of atoms, is bombarded with flowing energy (such as concentrated currents of electricity or heat), that system will often self-organize into a more complex configuration—specifically an arrangement that allows the system to more efficiently dissipate the incoming energy, converting it into entropy.
This basic process was described almost a century ago by the Belgium chemist Ilya Prigogine, and he called the emergent system a “dissipative structure.” A basic example of a dissipative structure is the tiny whirlpool that appears when you remove the stopper from a full sink or bathtub. The emergent vortex is better at dissipating the kinetic energy from the flowing water compared to when the water flows directly.
While Prigogine had some of the mathematics worked out for describing this self-organization process, it wasn’t until this decade that real progress was made in formalizing the phenomenon quantitatively. In a series of papers published over the years in various academic journals,
In two recently published computer simulation studies, one in the journal Proceedings of the National Academy of Sciences and the other in Physical Review Letters, England’s team showed exactly how a simple system of lifeless molecules, like those that existed on Earth before life emerged, may reorganize into a unified structure that behaves like a living organism when hit with a continuous source of energy for long enough. This occurs because the system has to dissipate all that energy, and biological systems, which must metabolize energy to function through chemical reactions, provide a way to do just that. The simulation studies visually depict how such a complex system can emerge from simple molecules when energy flows through that matter, much like the whirlpool that inevitably emerges in a draining sink. Organization results from a need to disperse energy. While England’s abstract digital representation of the process might not be a perfectly accurate description of how real chemical networks self-organize, since simulations are simplifications of real systems, other experiments that actually used physical materials have demonstrated the same fundamental process.
In a 2013 experiment published in the journal Scientific Reports, a group of researchers from Japan showed that simply shining a light (a continuous source of energy) on a group of silver nanoparticles caused them to assemble into a more ordered structure—specifically one with the ability to capture and dissipate more energy from the light. In a similar fashion, a 2015 study published in the journal Physical Review E demonstrated dissipative adaptation in the macroscopic world. When conduction beads were placed in oil and struck with voltage from an electrode, the beads formed intricate collective structures with “wormlike motion” that persisted as long as energy flowed through the system. The authors remarked that the system “exhibits properties that are analogous to those we observe in living organisms.” In other words, under the right conditions, hitting a disordered system with energy will cause that system to self-organize and acquire the properties we associate with life.
Though dissipative adaptation occurs before a system has genes, the basic chemical system can still evolve through a kind of primitive natural selection process that is easy to conceptualize. When a molecular system is undergoing natural fluctuations whereby its collective form is randomly sifting through a number of successive structural states, those arrangements that allow the system to more effectively extract energy from the environment—a requirement for survival—will persist, while those arrangements that do not go by the wayside. This is presumably how an inanimate network becomes a biochemical network, such as that of a cell.
While England’s simulation studies and the analogous physical experiments clearly show that there is a type of thermodynamic evolution that occurs before Darwinian evolution can kick in, the precise evolutionary stages between these types of emergent complex structures and living cells with genetic material is still unknown, as are the exact conditions on Earth that set the stage for dissipative adaptation. However, scientists from the Santa Fe Institute, the mecca of complexity research, have a pretty good idea of how it might have happened.
The emergence of life on Earth
Although England’s model of dissipative adaptation describes the mechanism through which complexity can spontaneously emerge as a result of energy flowing through lifeless molecules, it does not address how or why such a process happened on Earth. What sort of terrestrial environment allowed energy flows to create order from molecular chaos?
In the book The Origin and Nature of Life on Earth, published in 2016 by Cambridge University Press, physicist Eric Smith and biochemist Harold Morowitz theorize that life on Earth first emerged due to inanimate matter being driven by energy currents produced by the planet’s geothermal activity, like that which occurs in volcanoes and inside the Earth’s core. In their view, life was an inescapable consequence of free energy buildup, presumably in hot areas like the hydrothermal vents at the bottom of the ocean. It is in these types of regions where energy flows are concentrated enough to produce lasting chemical order.
Under this explanation, life formed as a sort of channel for relieving the energy imbalance through more efficient dissipation, and was “as inevitable as water flowing downhill.” And just as water flowing downhill carves a channel into the hillside that becomes progressively deeper with time, flows of energy reinforced and strengthened the chemical channels that we call metabolic pathways. Living things are just a better way for nature to dissipate energy and increase the universe’s entropy.
However, according to Smith and Morowitz, the self-organizing process that led to life didn’t occur in one step, but instead involved a series of what physicists call “phase transitions.” A phase transition is a fancy term to describe a holistic change in the overall arrangement of a system’s structure, and in turn, its function. Most people are familiar with the basic phase transitions that are taught in high school chemistry, such as how liquid water freezes into ice under cold temperatures. During this phase transition, the H2O molecules are transformed into a more stable configuration, from a liquid phase to a solid one.
This doesn’t only occur at the molecular level. A more complicated example is when flocks of birds fly in unison, forming shapeshifting geometric patterns that seem to constrain and partially guide the pack, a phenomenon that is stunning to watch. We can even think of the emergence of human civilization as a phase transition, in which chaos collapsed into order. Essentially, phase transitions are how new levels of emergence suddenly come into existence.
The series of phase transitions that led to life consisted of multiple jumps to more complex (and therefore stable) arrangements, in particular those system configurations that were better at relieving free energy build up through greater dissipation. Like England, Smith and Morowitz—who sadly passed away just before the publication of his opus—describe a natural selection process whereby those phases that best stabilize the system are the ones that endure.
This perspective helps explain why life is so robust and resistant to breakdown. As inanimate aggregates of particles evolved into chemical networks, and chemical networks evolved into simple information networks, the complex adaptive system became increasingly resilient and compartmentalized. With the birth of information systems—cells with genetic material and proteins capable of constructing the structures specified by the blueprints—self-replicating units, or “replicators,” were born, and biological evolution became possible. It was through this cascade of phase transitions that life emerged.
At home in the universe
By accepting dissipative adaptation as a spontaneous physical process, we also accept a new cosmic picture—one in which biology is fundamental. The laws of physics and the dynamics of nature not only allow for life, they necessitate it. The second law of thermodynamics does not just generate disorder; it is also a motor for complexity, because complex adaptive systems efficiently dissipate free energy, thereby increasing the universe’s entropy.
Despite these theoretical advancements, we still don’t know a lot about how life first emerged on Earth. But we do know that it arose relatively quickly (at least on a cosmic timescale—less than a half billion years after the planet formed), and that it has maintained its existence uninterrupted for almost 4 billion years, despite nature’s extreme turbulence. If life occurs inevitably as a result of energy flows driving chemical systems away from thermal equilibrium and into increasingly complex arrangements, that would have important implications for the possibility of life elsewhere in the universe.
For example, any calculations regarding the probability of alien life would have to take dissipative adaptation into account to be accurate. This would certainly increase the likelihood of life on planets with free energy reservoirs and conditions sufficiently similar to those on Earth. While on the surface this may appear to make the Fermi paradox even more perplexing, perhaps it can help explain it. If life emerges inexorably as a consequence of thermodynamics, then we might expect biospheres to be emerging and evolving on similar timescales. If this is the case, intelligent life originating on other planets simply may have not had time to interact with us. Additionally, dissipative adaptation speaks to only the emergence of biological life, not intelligent life, so it says little if anything about the likelihood of technologically-advanced extraterrestrials.
Sean Carroll, a theoretical physicist at the California Institute of Technology and author of The Big Picture, says that the question “Is life improbable?” can be interpreted in different ways, complicating the issue. On one hand, it can be a question of whether it is natural for life to occur in the universe as we know it, which seems to be the case given the mechanisms that have been described. It can also be a question about whether or not the physical parameters of our universe need to be fine-tuned to allow for life. In other words, does our universe hold some sort of special status among all possible universes? “It’s at least conceivable that life would have been much less likely if the laws of physics were just a bit different,” Carroll told Quartz.
While the verdict is still out on that one, in the context of the universe we find ourselves in, humans no longer need to think of themselves as a “cosmic accident.” If biological systems are a regularity, then we are in fact “at home in the universe,” as complexity scientist and author Stuart Kauffman has put it. And that is a thought that even an atheist can find spiritual satisfaction in.