The world has been clamoring for a super-battery.
Since about 2010, a critical mass of national leaders, policy professionals, scientists, entrepreneurs, thinkers and writers have all but demanded a transformation of the humble lithium-ion cell. Only batteries that can store a lot more energy for a lower price, they have said, will allow for affordable electric cars, cheaper and more widely available electricity, and a reduction in greenhouse gas emissions. In the process, a lot of gazillionaires will be created.
But they have been vexed. Not only has nobody created a super-battery; a large number of researchers have lost faith in their powers to do so—perhaps ever. Entrepreneurs such as Tesla’s Elon Musk continue to tinker with off-the-shelf batteries for luxury electric cars and home power-storage systems, but industry hands seem generally to doubt that their cost will drop enough to attract a mass market any time soon. Increasingly, they are concluding that the primacy of fossil fuels will continue for decades to come, and probably into the next century.
This is where Yet-Ming Chiang enters the picture. A wiry, Taiwanese-American materials-science professor at the Massachusetts Institute of Technology (MIT), Chiang is best known for founding A123, a lithium-ion battery company that had the biggest IPO of 2009. The company ended up filing for bankruptcy in 2012 and selling itself in pieces at firesale prices to Japanese and Chinese rivals. Yet Chiang himself emerged untainted.
In 2010, having rounded up $12.5 million from Boston venture capital firms and federal funds, Chiang launched another company. Again, it was in batteries. And today, after five years in “stealth mode,” he is going public. There may be a way to revolutionize batteries, he says, but right now it is not in the laboratory. Instead, it’s on the factory floor. Ingenious manufacturing, rather than an ingenious leap in battery chemistry, might usher in the new electric age.
When it starts commercial sales in about two years, Chiang says, his company will slash the cost of an entry-level battery plant 10-fold, as well as cut around 30% off the price of the batteries themselves. That’s thanks to a new manufacturing process along with a powerful new cell that adds energy while stripping away cost. Together, he says, they will allow lithium-ion batteries to begin to compete with fossil fuels.
But Chiang’s concept is also about something more than just cheaper, greener power. It’s a model for a new kind of innovation, one that focuses not on new scientific invention, but on new ways of manufacturing. For countries like the US that have lost industries to Asia, this opens the possibility of reinventing the techniques of manufacture. Those that take this path could own that intellectual property—and thus the next manufacturing future.
This is the story of how that came about.
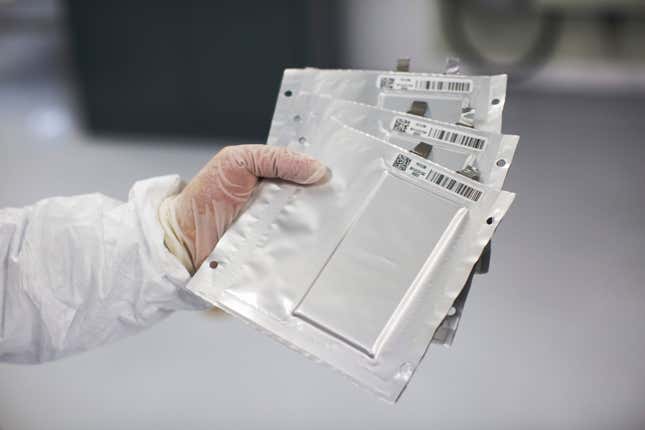
Manufacturing, the new frontier of innovation
Traditionally, big innovations have happened at the lab bench. A discovery is made and patented, then is handed off to a commercial player who scales it up. With luck, it turns out a blockbuster product.
But, according to a report published in February by the Brookings Institution, researchers are increasingly skeptical of the delineation between innovation and production. Breakthrough-scale invention, they say, happens not only in the lab, but also in factories.
This is not a new idea. Until 1856, for instance, steel was an ultra-expensive niche product. It was far more robust than iron, but no one knew how to make it economically. Its use was confined to specialty hand tools and eating utensils for the rich. But then British inventor Henry Bessemer, stirred by French gripes about the fragility of cast-iron cannons, devised a process that reduced the cost of steel by more than 80%, roughly equivalent to iron. Steel—along with oil—went on to propel the latter part of the Industrial Revolution, along with the gargantuan 20th century economic boom.
If Bessemer had made his breakthrough today, it would be called “advanced manufacturing”—a label that has been broadly applied to next-generation fabrication methods such as 3D printing, modular construction of skyscrapers, and robotics. There is some hype around this term: The Brookings report identifies 50 industries in the US alone as “advanced,” and historic factory hubs such as the English city of Sheffield are renaming themselves as variants of “advanced manufacturing cluster.”
Nonetheless, entrepreneurs who develop genuinely novel manufacturing processes can enjoy the advantage of a patent and standing ahead of the crowd. While others will inevitably copy them, it will be a race to catch up. To the degree that such authentic advanced manufacturing moves forward, and can offer the US a chance to reinstate its prowess as a manufacturing hub, it’s led in part by a few clean energy companies like Yet-Ming Chiang’s.
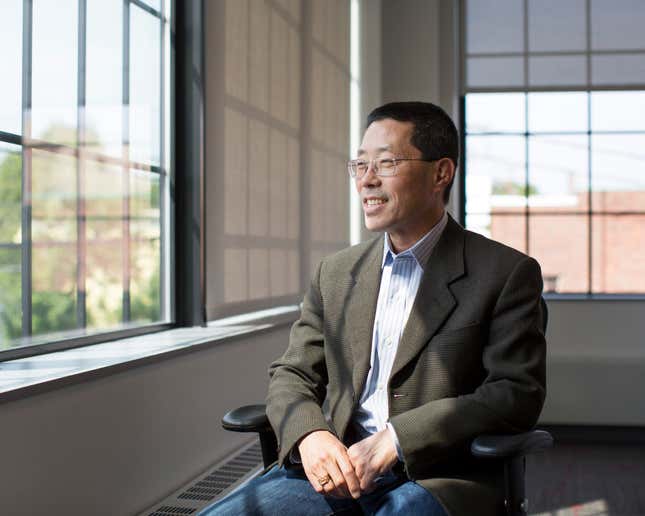
The birth of an idea
At 57, Chiang has short-cropped, gray-flecked black hair, and almost always wears blue, long-sleeved check shirts. He speaks in a soft, even cadence, and is prone to finishing his sentences with a disarming, open-jawed grin.
But if unassuming, Chiang is also tremendously driven. His science-centered business sense has earned tens of millions of dollars for his investors. He and his family live on a farm on the affluent outskirts of Boston, where he raises bees and chickens, and hunts and fishes nearby.
Chiang was born in Taiwan, where his father, a locomotive engineer, managed to save enough money to make a start in the United States. When the boy was 6, he found himself in Brooklyn, living with his family in an apartment with what he regarded as astonishingly high ceilings. When it was time for college, Chiang was admitted to MIT, and never left. His wife, Jeri, a Japanese-American from Hawaii, also has an MIT degree, as do his older sister and her husband.
Like Stanford University now and the University of Copenhagen in the 1920s, MIT is a maw of discovery and celebrity scientists.Chiang calls it a “meritocracy”—a “praise-free zone” where “you are what you do and what you create. You should continue to try to prove yourself.” Chiang has used his own MIT perch to launch four venture-capital-funded startups, including his latest, a battery company called 24M.
Manufacturers are secretive, but analysts say a lithium-ion battery pack costs an average of roughly $500 per kilowatt-hour, a measure of the energy a battery can store. That’s four times the price needed to compete directly with gasoline. Only about 30% of that $500 is the cost of materials. The largest portion, 40%, goes to manufacturing.
Battery factories themselves are typically cavernous buildings the size of aircraft hangars. They contain assembly-line machines dozens of yards in length, often stacked one atop the other. The cost for an entry-level plant is more than $100 million. In Midland, Michigan, XALT runs one of the most efficient and modern lithium-ion plants in the US. But, built with $300 million in federal and state grants and credits, it is also sprawling—just under a quarter of its 400,000-square-foot (37,000 sq m) facility is devoted to the equipment, a space the size of six soccer fields. Tesla is embarked on the mother of battery plant buildouts, a $5 billion lithium-ion factory in Nevada.
Such costs not only make batteries expensive. They also stifle innovation. Who, even with a promising new idea for a better battery chemistry, can build or borrow a $100 million plant to try it out?
Chiang’s goal is to bring production costs down below $100 a kilowatt-hour. That would allow startup plants to be built for much, much less, unleashing innovation. And it would also create a genuine contest with gasoline.
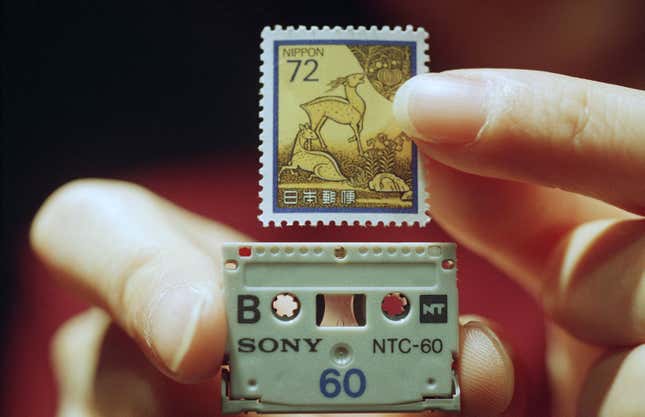
The battery’s ungainly legacy
The reason battery factories are so huge—and why Chiang’s business model seems to have substance—goes back to a chance event at the birth of lithium ion.
The rise of lithium-ion chemistry in the early 1990s owes a lot to the peak and slow decline of two big consumer technologies—magnetic audio tape and nickel cadmium batteries. These two collided in the Camcorder, Sony’s entry into the nascent market for lightweight video cameras.
Sony realized that, if video cameras were to take off, they needed both to shrink—to more or less fit snugly into a consumer’s hand—and to last longer on a single charge. The only way to accomplish that was to find a far more powerful, smaller battery.
The result was the first lithium-ion cell, which Sony commercialized in 1991. Two years later followed the TR1 8mm Camcorder, the first lithium-ion-operated video camera. Both were blockbuster commercial products for Sony, and ignited furious competition.
But Sony also had to quickly figure out how to manufacture this new kind of battery on a commercial scale. Providence stepped in: As it happened, increasingly popular compact discs were beginning to erode the market for cassette tapes, of which Sony was also a major manufacturer. The tapes were made on long manufacturing lines that coated a film with a magnetic slurry, dried it, cut it into long strips, and rolled it up. Looking around the company, Sony’s lithium-ion managers now noticed much of this equipment, and its technicians, standing idle.
It turned out that the very same equipment could also be used for making lithium-ion batteries. These too could be made by coating a slurry on to a film, then drying and cutting it. In this case the result isn’t magnetic tape, but battery electrodes.
This equipment, and those technicians, became the backbone of the world’s first lithium-ion battery manufacturing plant, and the model for how they have been made ever since. Today, factories operating on identical principles are turning out every commercial lithium-ion battery on the planet.
For Sony, the idle magnetic tape machines were a piece of good fortune. But Chiang regarded them as an ungainly legacy. The machines were big, and their process was slow and expensive. They were a large part of the reason batteries couldn’t compete with gasoline. It was time to correct that mistake and figure out a new way to make the battery. “We got sidetracked by a historical accident and a reluctance to switch to something that works (better),” Chiang said.
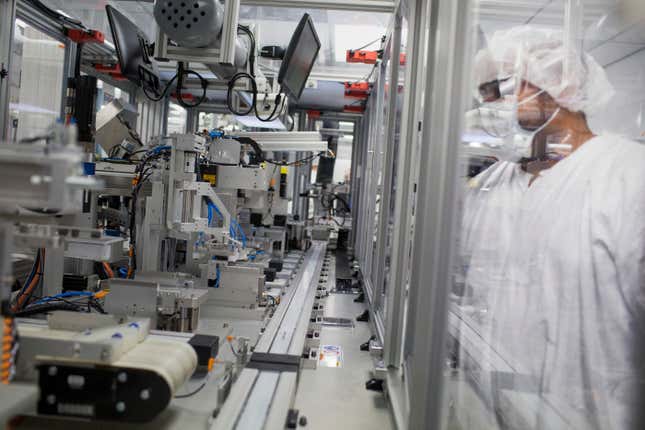
Going with the flow
At first, Chiang thought the best solution was an arcane and eccentric technology known as a “flow battery.” His interest flummoxed many of the people he talked to.
A battery is superficially fairly simple. It essentially consists of two electrodes, which are the source of the electric charge, embedded in an electrolyte, through which the charge flows. In a conventional lithium-ion battery, the electrodes are solids, all stored in a single cell or pack.
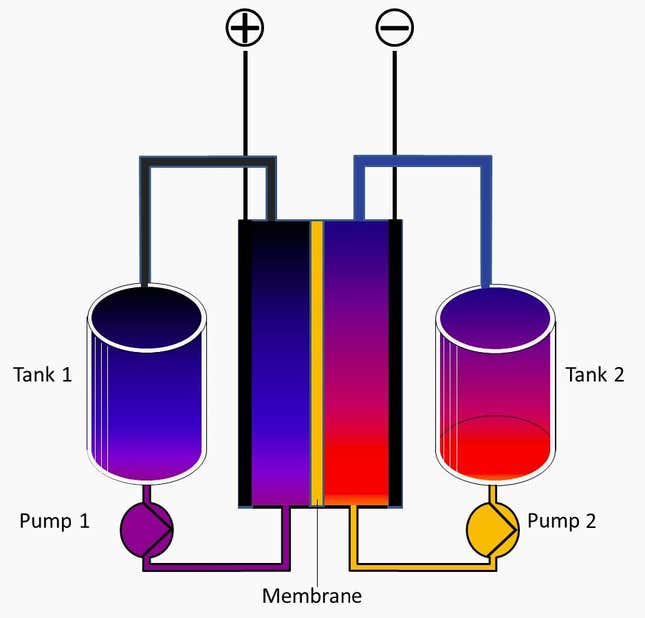
A flow battery, by contrast, consists of chemicals suspended in liquid. This liquid is held in two separate tanks, from which they are pumped through a cell. There they meet, separated by a membrane. The act of pumping them generates a current that flows between them across the membrane.
To increase the capacity of a battery, you need to either boost its energy density, or make it bigger. For lithium-ion batteries, increasing the energy density—by tweaking the battery chemistry or finding a new kind—is the holy grail scientists are starting to despair of ever finding. Making them bigger is easy; Tesla has done just that for its cars. But they get expensive fast, because they require more of the costly metals, like nickel and cobalt, that go into the electrodes of lithium-ion cells.
By contrast, making a flow battery bigger is just a matter of bolting on larger storage tanks with more liquid inside. But the device would quickly become far too big to fit inside a car, and the liquid chemicals in a flow battery have a much lower energy density than a lithium-ion battery.
But what if you could have the best of both worlds? That was the original thesis of Chiang’s new venture. If you could make a flow battery with lithium-ion chemistry—and its energy density—it would have smaller tanks than a regular flow battery. Above a certain size, the cost per kilowatt-hour would be below that of static batteries, and begin to compete with the economics of fossil fuels.
At MIT, Chiang assigned a Romanian undergraduate named Mihai Duduta to study the problem. A month later, Duduta had a working prototype. The rapid result was a surprise, and also evidence that Chiang was on to something. It was sufficient to attract $10 million in funding from Boston venture capital firms, and another $2.5 million from the Department of Energy. With that, Chiang opened 24M for business. Duduta was employee No. 1.
The company was operating in stealth mode, so little was released publicly. But in a 2011 paper in the journal Advanced Energy Materials, Duduta explained an order-of-magnitude increase in energy density through a “semi-solid” approach to flow—a lithium-ion battery that worked through “percolating networks of nanoscale conductors.” Now, as far as the world was concerned, Chiang’s latest startup was a quixotic hunt for a world-beating flow battery.
But that would soon change.
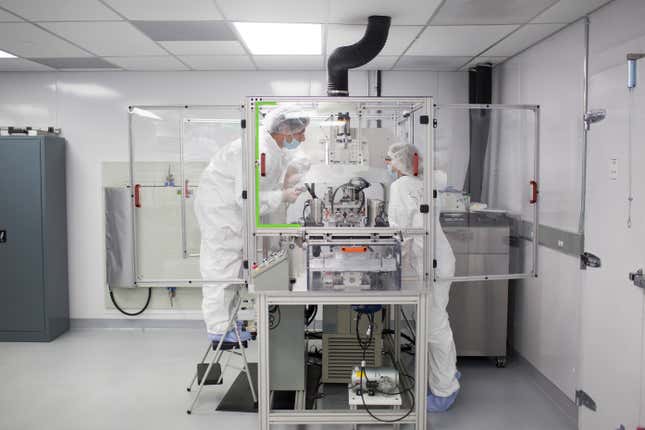
An economic quandary
The success or failure of Chiang’s idea was in part a function of size. How big would the tanks of lithium-ion flow batteries need to be in order for their cost per kilowatt-hour to drop below that of static batteries?
By late 2010, this problem weighed on Craig Carter, Chiang’s long-time collaborator at MIT. When original 24M employees gathered for weekly meetings to parse their data, no one seemed to know what size tanks, test cells and other equipment to buy and make. The cost model they were using did not make it clear enough when the economic crossover from static batteries would occur.
That wasn’t the only problem 24M was facing. Nobody had ever made a lithium-ion flow battery. Chiang’s engineers were having trouble figuring out how to pump the electrolyte liquid through the system. The denser they made the slurry, to increase its energy density, the thicker and more sluggish it became. Potential customers, after being briefed by senior executives, offered little encouragement. Conventional static batteries already worked fine; why did anyone need a new kind of lithium-ion battery that also required a pump?
Meanwhile, a side experiment within 24M was starting to attract the attention of Chiang’s junior researchers. For comparison purposes, Chiang had instructed them to create static lithium-ion cells alongside the flow project. “We can learn from them,” he said. The results were interesting: the team had used the same liquid slurries as they had in the flow battery to make hundreds of static cells, and they put them through thousands of charge-discharge cycles. Their capacity remained stable. Unlike the flow experiment, they worked superbly.
After work, some of the junior staff including Duduta would troop downstairs for milkshakes at an eatery called Friendly Toast. There, they discussed the results from the static cells. These younger researchers were less invested than Chiang and the senior staff in the idea of flow, recalled one of them, Tristan Doherty, a former race engineer for Dale Coyne’s Indy 500 racing team. Gradually they became convinced that the new manufacturing process they were developing should be devoted to making static, not flow, batteries. But how to get that message across to their elders?
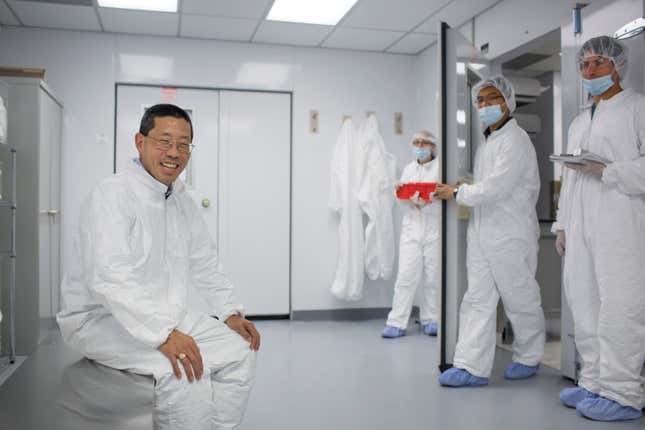
The moment when it all fell apart
It was in this environment that Carter was trying to figure out at what point flow batteries would become economical. Chiang did not seem to think it was a problem. “You may be wasting your time,” he told Carter. Carter persisted, and finally decided to put aside the cost model they were using and build his own. He enlisted one of the young staff—Jeff Disko, a Wyoming native who favors cowboy boots and self-carved silver belt buckles. “Let’s build it from scratch,” Carter told the younger man.
What he didn’t do was tell Chiang what he and Disko were up to. “He might have seen it as a distraction from going forward since we already had a working tool,” Carter said.
Disko worked around the clock for two weeks on the data while Carter created software that could visually display almost any battery variable—energy density, speed of charge, cost of parts, and so on.When they were done, they had a tool that finally revealed the crossover point at which Chiang’s battery would prove economical.
To say it would require enormous tanks would be an understatement. To be competitive with fossil fuels, a lithium-ion flow battery would have to be large enough to back up a stationary facility the size of a nuclear power plant serving tens of thousands of people. It was such a jaw-dropping result that neither Carter nor Disko believed it initially. They spent two weeks redoing the numbers and discussing the results. Disko began to vet it with the rest of the group. But there was no getting around it—the idea on which the company had been founded did not make financial sense.
In early 2011, they held what Disko called a “come-to-Jesus meeting.” He presented the visual tool. Until then, there had been the grumblings, but no brutally concrete juxtaposition of flow and static batteries. Now it seemed clear—unless you were aiming to back up the electricity system of a small city, it was better to build a static battery.
Chiang stared at the results. “So are you willing to bet the company?” he asked Carter.
“Yes,” Carter replied.
“Okay,” Chiang replied simply. He would think about it.
Two days later, an email went to all employees. Flow was out. The company would build a static battery.
It was a typical shift for a startup, in which initial notions rarely survive through the commercial stage of development. For his part, Disko felt “relieved. I think a lot of people did.” The manufacturing problems still needed to be solved. But now they would attack them differently. The cost model had proven its value. “There are benefits of changing direction—of turning on a dime,” Carter said. “Now we had something in which you could plant a flag and know it would stick.”
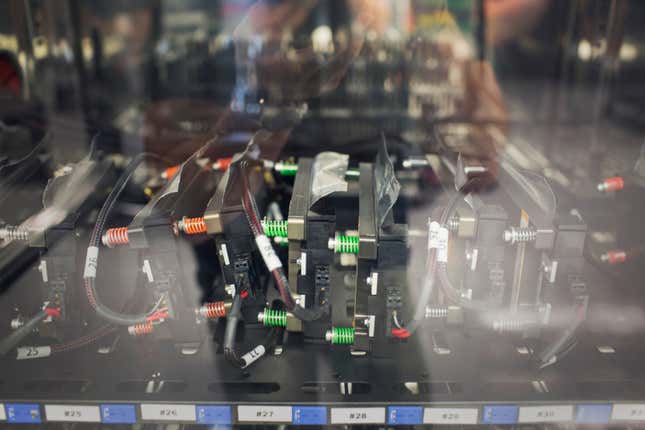
Starting from zero
Now the researchers could return, metaphorically, to the age of the Camcorder and pose the question: If Sony hadn’t had those magnetic tape machines lying around and had started from a blank slate, what would have been the most natural and best way to manufacture a lithium-ion battery?
Pumps intended to initiate the flow of electron juice started to disappear from the 24M lab. Then Duduta, the conceiver of the original 24M flow cell, waited a few weeks, before declaring, “I am going to make these [static] cells myself.”
There was no machine for this task, so Duduta stuck his arms into the black rubber gloves of an airless research box—known as a glove box—and began to hand-make cells. That meant mixing up the goop, or slurry, that comprises the two electrodes—the anode and cathode—and slapping them onto a thin film, separated by another plastic film.
A couple of the others joined him. Soon, six or seven researchers had their hands in the rubber gloves. They had created their own manual assembly line. They became good at it—they were producing automobile battery-size cells in just six minutes. Compared to the day-long process required in a conventional factory, that was lightning fast. But it was nothing to the speed with which Chiang would eventually want the process to go.
In the conventional process, the application of the slurry is relatively quick, but the drying stage can take 22 or more hours. You start out with wet slurry, then coat it onto film—using glue-like substances to make it hold—press it flat to make the electrodes denser, and finally dry it in an oven along the long, slow assembly line. Finally, electrolyte is injected into the battery cell, thus making it wet all over again.
Apart from this slow process, conventional batteries have a second problem: 35% of their interior space is filled with material that doesn’t contribute to generating electricity. That includes the binder that holds the slurry to the film; a separator that keeps the anode and cathode from shorting each other out; and a current collector that brings the charge to an electronic device.
Chiang wanted to reduce the manufacturing process to a single hour. And he wanted to shrink the space filler to almost nothing.
He started out by whacking out whole parts of the filler. His researchers developed a way to make the electrodes without the glue-like binder. Lithium-ion cells typically contain 14 separate material layers; Chiang simplified them, allowing him to reduce the layers to just five. He reduced the filler to 8% of the battery cell. Finally, he overturned the foundations of lithium-ion manufacturing by figuring out how to dispense entirely with the drying process; instead, he would inject the wet electrolyte into the cell from the start.
These were defining improvements. But, while he was at it, Chiang made some tweaks to the science of the battery, too. Most significantly, he made the electrodes four times thicker—500 microns, or half a millimeter, in diameter—which added a lot to the cells’ energy density.
Still, there was the matter of how to actually get the wet electrode slurry onto film in a uniform density, thickness, continuity and rectangular shape, and to do so fast and in a way that could be replicated over and over again.
Some three dozen ways were attempted to get the slurry right. The final method was “a total shot in the dark,” Doherty said, and involved a tube, a plunger, and some Teflon.
But the result was a manufacturing platform that currently spits out a battery cell in about two and a half minutes. The machine that does it isn’t the size of a factory floor, but of a large refrigerator (see image below). As for the cells, Chiang calls them “semi-solid,” a nod to their birth in research into flow batteries.
When I was visiting their lab recently, Chiang and 24M’s CEO, Throop Wilder, stood around the machine as it spit out a fast cell in a perfect rectangle. Wilder started doing jumping jacks. “That’s huge. That’s what investors want to see,” he said, shouting.
Chiang’s sudden pivot to static batteries doesn’t appear to have unnerved 24M’s investors. In 2013, Chiang raised another round of $25 million in cash, and last year PTT, the Thai oil company, invested $15 million. In all, 24M has raised $54.5 million. “They are able to introduce a novel battery that’s 50% like bringing the economics of Moore’s Law into an industry that doesn’t have that,” said Izhar Armony of Charles River Ventures, one of Chiang’s VC investors.
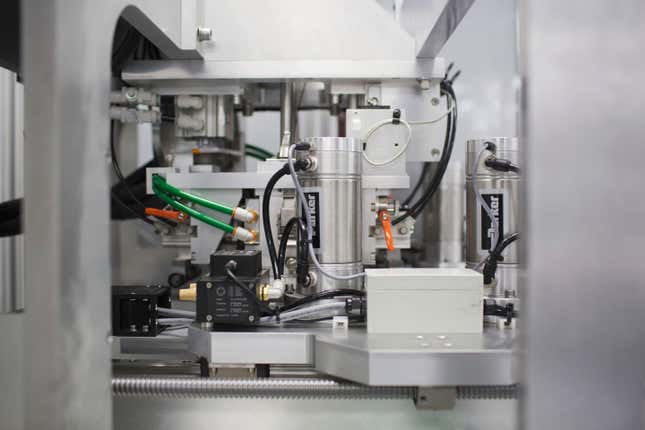
What it means for manufacturing
The push to improve the manufacturing, as opposed to the chemistry, of lithium-ion batteries has caught on with US government officials. The Department of Energy (DOE) is currently running a competition for three-year grants worth $6 million to $8 million for researchers promising better manufacturing techniques. If they can make such progress, and add it to any advance on the scientific side, “then you’ve double-dipped,” said David Howell, who runs the DOE battery research program. Howell said that is how he expects to make electric cars equivalent, in dollars per kilowatt-hour, with gasoline.
The advanced manufacturing movement has spread to solar as well. Frank Van Mierlo, founder of a Massachusetts solar panel company called 1366, said that his own industry’s standard manufacturing method “is reminiscent of how the Greeks made glass.” He said his company has devised a new way to make panels that chops out much of the inefficiency.
In a new report, McKinsey describes a broad new age of manufacturing that it calls Industry 4.0. The consulting firm says the changes under way are affecting most businesses. They are probably not “another industrial revolution,” it says, but together, there is “strong potential to change the way factories work.”
For decades, the US has watched its bedrock manufacturing industries wither away, as they’ve instead grown thick in Japan, in South Korea, in China, Taiwan and elsewhere in Asia. According to the Economic Policy Institute, the US lost about 5 million manufacturing jobs just from 1997 to 2014. This includes the production of lithium-ion batteries, which, though invented by Americans, were commercialized in Japan and later South Korea and China.
So Chiang’s innovation could be a poster-child for a new strain of thinking in the US. This says that, while such industries are not likely to return from Asia, the US can possibly reinvent how they manufacture. The country wouldn’t take back nearly as many jobs as it has lost. But there could be large profits, as the country once again moves a step ahead in crucial areas of technology.
To be clear, this is not Chiang’s goal. He is a professed universalist, divorced from scientific realpolitik. But should he succeed, as he plans to, then in addition to helping to decode the perplexing problem of batteries, he might contribute to continuing America’s political and economic dominance.
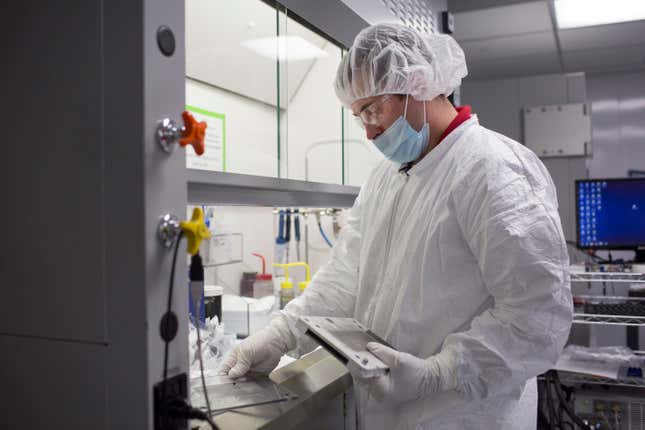
The road ahead
Chiang and Wilder are about to embark on a third round of investment, seeking $20 million to $30 million. They would spend the money to scale up to production of a new machine that makes a cell every two to ten seconds. This machine, to be available for sale in two years, would be for stationary electric batteries—used to power businesses, neighborhoods and utilities, rather than cars.
The machine would have a capacity of 79 megawatt-hours a year and produce any kind of lithium-ion battery for a cost of about $160 per kilowatt-hour. By 2020, Chiang says, that will be down to about $85, 30% below where conventional lithium-ion batteries—whose cost is also dropping—may be by then. But most importantly, the machine would be priced at about $11 million. Hence, the startup cost of getting into lithium-ion battery manufacturing would plummet. “It’s so far out of the paradigm, you just don’t believe it,” said Wilder.
If 24M creates this machine, and if it can sell it into the market—an entirely different question—it will clearly shake up big industries, including stationary and electric car batteries, not to mention utilities. How quickly is anyone’s guess.
Chiang seems ambivalent as 24M begins to disclose what it’s been doing all these years. Until now, the entire industry has had a singular idea of how batteries are manufactured. Chiang’s own rivals were, until today, convinced that he was on a far-fetched crusade to figure out flow batteries.
But now, if they look hard at what he is really doing, and accept his approach, they may attempt to copy him. “If you haven’t seen the movie play out before, you don’t have the confidence it can be done,” he said. But staying a step ahead is also part of the startup game.