2015 was the year it became OK to genetically engineer babies
In April of this year, researchers in China published the results of an experiment in modifying the DNA of human embryos. Though the embryos they worked on were damaged ones that could not have grown into living babies, it sent a tremor through the scientific establishment.
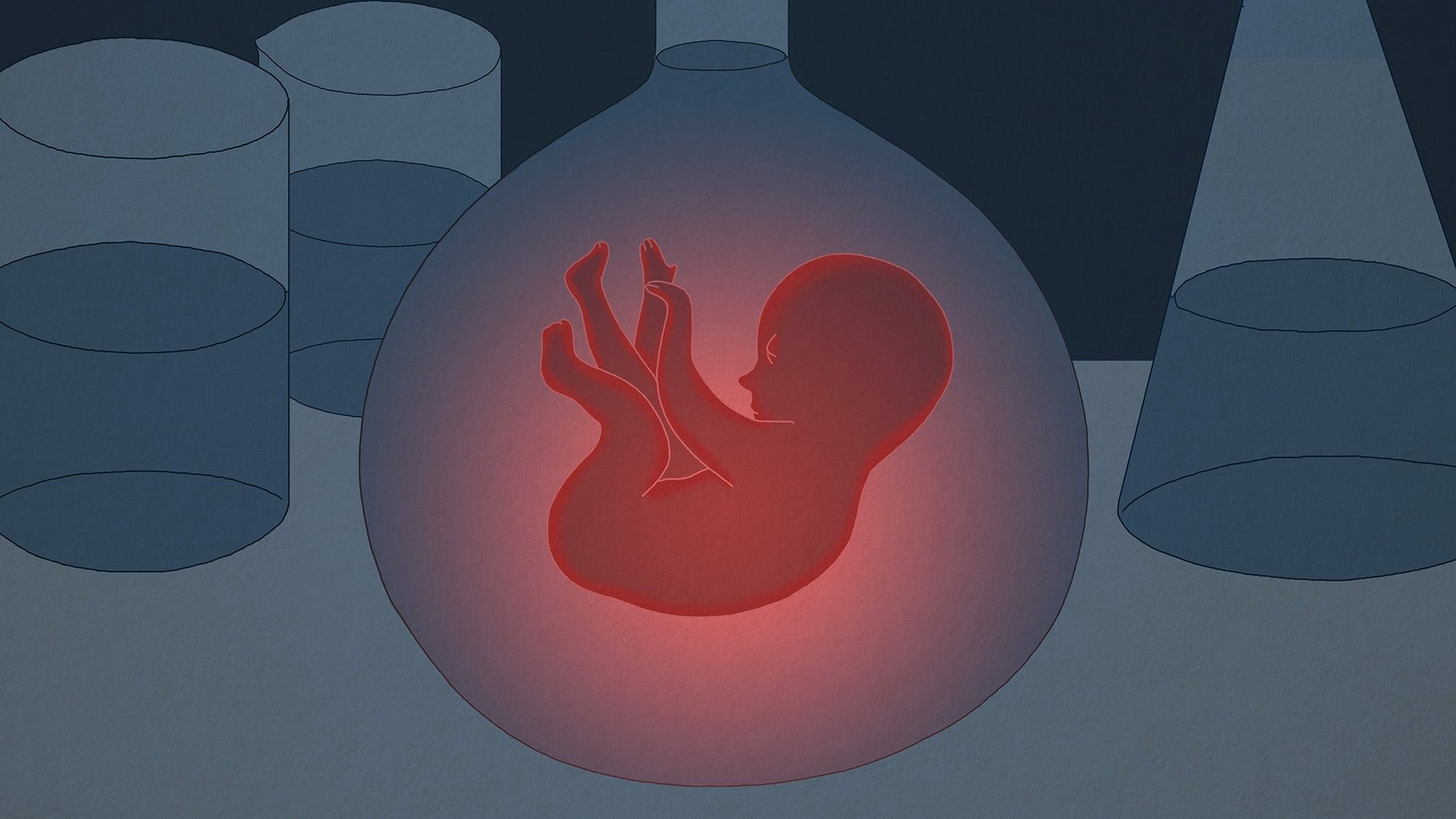
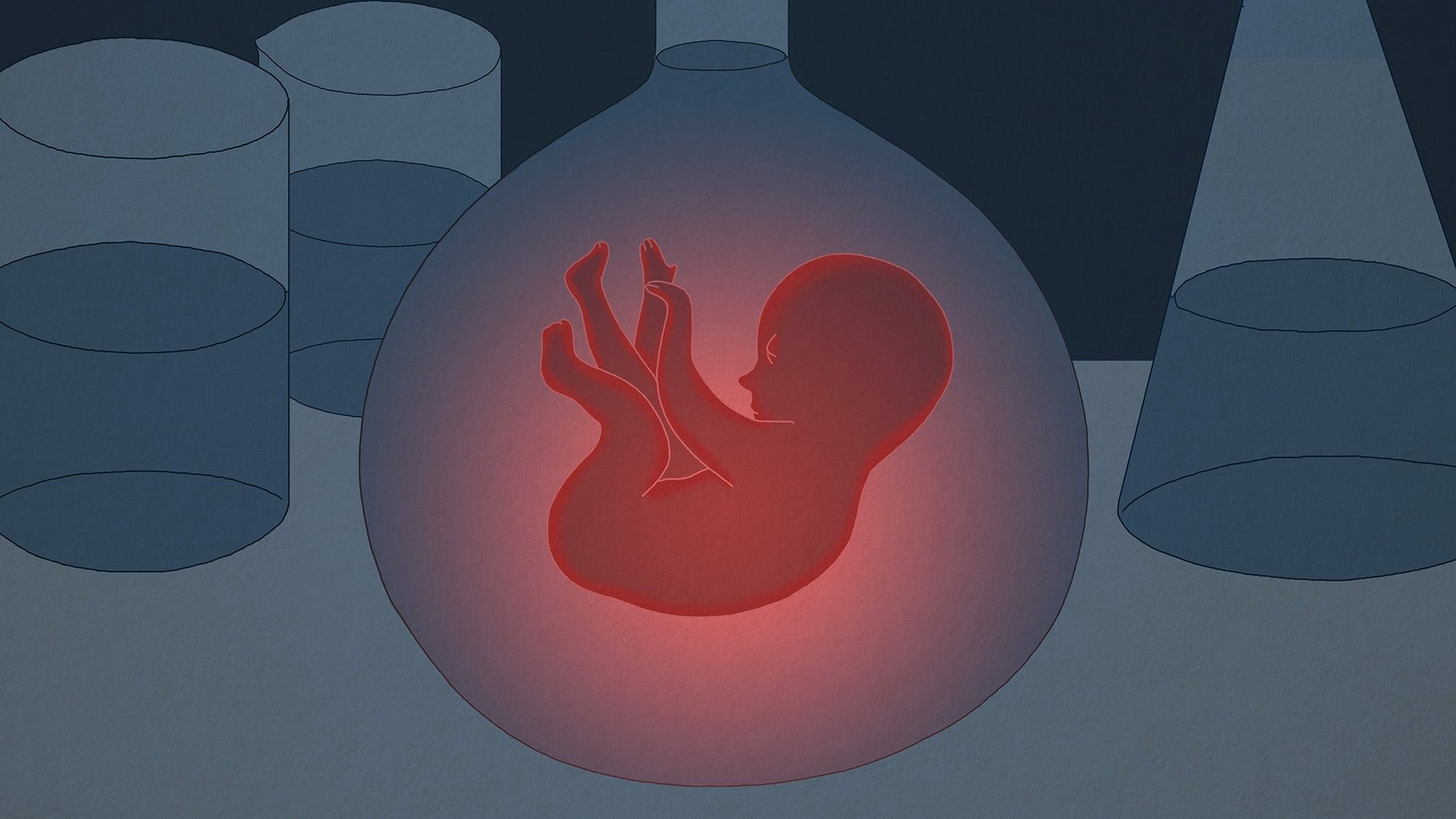
In April of this year, researchers in China published the results of an experiment in modifying the DNA of human embryos. Though the embryos they worked on were damaged ones that could not have grown into living babies, it sent a tremor through the scientific establishment.
Just a month earlier, a group of leading geneticists had called for a moratorium on gene-editing in embryos. Since any genetic changes would get passed on to future generations, they argued, the risk of unintended consequences was too great. And in November, at a summit in Washington DC, scientists from across the world agreed that, while research should continue, it’s still too risky to allow any altered embryos to grow into full human beings.
And, yet, when historians of science look back decades from now, they may well mark 2015 as the year genetically engineering humans became acceptable. That’s because, while the world was paying attention to the gene-editing summit, a more momentous decision had been made just a month earlier in the UK. There, a governmental body got ready to hand out licenses for creating a particular kind of genetically engineered human—using a technique the US tried and then banned 13 years ago.
This technique won’t create the fabled “designer babies” just yet. But the changes made to an embryo will be hereditary, and thus alter the genetic makeup of all the offspring to follow. The story of how we got here, and what will come next, is why 2015 will be remembered as a turning-point.

Just getting better
Our ability to do some form of genetic engineering goes back 40,000 years. Selective breeding created a tamer and more likable version of a wolf, the common ancestor of all today’s dogs.
Our desire to design better humans is also old. In Plato’s Republic, Socrates calls for a state-run program to get the best citizens to mate so that the population could be improved.
By the 19th century, the ideology of eugenics—a word not invented by Plato, but coined much later from the Greek for “good breeding”—had taken such a hold that countries were passing laws for such programs. Before World War II, 30 states in the US had passed some form of eugenics laws that mandated sexual sterilization of those deemed unfit (typically the mentally ill).
Only after the horror of Hitler’s genocide did the world recoil from eugenics. Most geneticists never returned to the idea that biological intervention would build a better society than social intervention. As Nathaniel Comfort, a professor of history of medicine, writes in Aeon, eugenics survived only in the form of ”preventive medicine for genetic diseases”—such as screening people for them and, occasionally, treating them with gene therapy.
Not everyone stopped trying. Taking inspiration from Aldous Huxley’s Brave New World, eugenicist Robert Graham created the Repository for Germinal Choice, a sperm bank for the super-intelligent. The bank existed from 1980 to 1999 and had some 19 high-IQ donors, including at least one Nobel laureate (William Shockley).
The resulting “Genius Babies ”—some 200 of them—are no different from normal people. One of those conceived through Graham’s sperm bank told CNN, “There’s only so much you can control when it comes to genetics. It all has to do with what you give to your family.”

Beyond our control
That comment defines the limits of science today. In the 1970s, we finally understood how to tweak the genes of microbes, plants and animals to achieve certain traits. But in humans, with the exception of a few things such as color blindness or tasting certain foods, “designer baby” traits, such as greater intelligence, taller stature, stronger muscles, or better memory, are controlled by hundreds of genes, each of which also perform many other critical functions. Tools that can deal with such complexity are still a long way off.
For now, then, the only foreseeable use for gene-editing is to prevent disease. And the goal is to make genetic tools good enough to do that without any unintended consequences.
Since 1989, thousands of people have received experimental gene therapies. Typically these involve the use of a “vector”—a biological vehicle, such as a virus, that can deliver the correct copy of a faulty gene to the specific cells in the body affected by the genetic disorder, such as cancer cells or faulty cells in the eye.
While most of these treatments have been safe, only a few have been effective. China approved the world’s first gene therapy in 2003 to treat certain kinds of cancers. Europe got its first in 2012 that treats a rare inherited disorder (pdf) affecting the pancreas. The US is likely to get its first gene therapy approved in 2016 to treat a form of blindness.
None of these therapies, however, have used the latest advance in gene-editing: CRISPR-Cas9, a highly precise copy-and-paste tool that allows for the removal and replacement of individual genes. Since its development in 2012, it has become an instant favorite among scientists.
CRISPR-Cas9’s immediate potential lies in curing single-gene disorders in embryos. Changes made at that stage would affect every cell in the body and could cure many diseases. We know some 4,000 such disorders, and, though each is rare, put together they could change the lives of millions in the next generation, and keep many more free from those diseases for all the generations to follow.
However, the Chinese study earlier this year showed that we might need something even more precise than CRISPR-Cas9 currently is. Only in one-third of the 86 embryos was the faulty gene erased as predicted, and even in those cases, CRISPR-Cas9 had also modified things it wasn’t meant to—the unintended consequences scientists worry about.
There is, however, another form of genetic engineering of human embryos. This is the one that the US tried and then banned, and that the British government recently opened licensing applications for. And what’s probably more important for the future of the debate is how Britain decided the technology works and is safe.

Three-parent child
Alana Saarinen was born in the US with three biological parents. Two of them contribute more than 99% of her genetic material and the third provides the rest. She is one of only 30 or so people in the world who grew from a genetically engineered embryo into a healthy adult.
Sharon and Paul Saarinen had attempted in-vitro fertilization (IVF) four times, but without success. A likely reason was that Sharon’s egg cells had faulty mitochondria. These are like biological batteries within a cell—they play an essential role in converting your food into the energy that powers your body. Uniquely, they also have their own DNA (though it’s only some 37 of the 20,000 or so genes that make up the human genome).
During reproduction, when an egg cell fuses with a sperm cell, creating the first cell of an embryo, it’s only the DNA in its nucleus that is a mix of both parents’ DNA. Mitochondria and their DNA are passed on directly from mother to offspring. Because Sharon Saarinen’s mitochondria were faulty, she basically needed a mitochondria transplant in order to conceive.
That was where the third parent came in. A donor provided an egg cell, whose nucleus was removed and healthy mitochondria along with other bits of the cell were transferred to Sharon’s egg. The egg cell was then mixed with Paul’s sperm cells in a normal IVF procedure, and the resulting embryo would become Alana Saarinen.
This technique won’t only help women like Sharon conceive. In a lot of cases with faulty mitochondria, pregnancies proceed normally, but the child then turns out to have one of several mitochondrial diseases, which can lead to all sorts of problems, from poor growth to autism to diabetes. One in every 5,000 children suffers from one of them.
Mitochondrial replacement therapy like the Saarinens had is currently the only known way of preventing mitochondrial diseases. But since they conceived Alana in 2000, only some 30 or so children have been born using the technique. In 2002, the US Food and Drug Administration (FDA) banned its use. Apart from ethical concerns about scientists “playing God,” there was a scientific worry too. We had never attempted to edit the “germ line”—the DNA that is transferred from one generation to another—and the risks were unknown. (About 10% of the pregnancies that resulted from this treatment had complications, but it wasn’t clear whether the procedure was to blame.)

Selfish genes
The FDA ban meant that US women with faulty mitochondria were left with difficult choices (pdf). They could choose not to have children, or undergo IVF and pick the fertilized embryo with the fewest defective mitochondria—taking a gamble on whether their child would develop mitochondrial disease.
But nearly a decade later the UK government’s Human Fertilization and Embryology Agency (HFEA) took up the case. In 2012, after taking a detailed look at the results of studies on animals and humans, it deemed that mitochondrial replacement therapy was “not unsafe”—meaning that the benefits of curing mitochondrial disease would outweigh the risks of the procedure.
The interesting thing was what the HFEA did next. In Sept. 2012, it launched a public consultation, creating a website that explained both the risks and benefits, and holding public events to do the same. Then it conducted a survey and asked people to send in their comments online. After the public had shown broad support for the therapy—and despite stiff opposition from scholarly groups and religious groups alike—the HFEA spent two years taking the necessary steps to get the regulations discussed in parliament. In February, MPs agreed to allow the use of the therapy under strict guidelines. In October, the process for handing out licenses began.
We already use genetic engineering to create climate-resistant crops and drug-producing bacteria. Now one of the world’s most scientifically advanced countries—and, fittingly, the birthplace of IVF—has agreed that genetically modified humans, too, are sometimes not just OK, but desirable. This is what makes 2015 an historic year.
Based on past progress, it is likely that genetic enhancements to humans will become a reality step by step. Just like mitochondrial replacement therapy, they will first appear for a very narrow purpose, such as curing single-gene disorders, and then, likely over many decades, we might reach the stage of creating those fabled designer babies.
That gives us enough time to deliberate the implications of each step. When our decisions will affect generations of humans to come, it is important we use that time well. The process that HFEA designed to win public and political support is a model worth emulating. If each step were to get the same scrutiny that mitochondrial replacement therapy got, genetically modified humans could become as normal as genetically modified crops and bacteria are today—and, barring the occasional controversy, as widely accepted.
Corrected Dec. 23: An earlier version of this post incorrectly said that Sharon Saarinen’s nucleus was implanted in a donor’s egg. It also said that HFEA began handing out licenses in October, but in fact it then began the process of handing them out.