I explore what’s possible in science by making molecules that shouldn’t exist in nature
At drinks parties and dinners, if someone asks what I do for a living, I always say: “Synthetic chemist … I make new molecules … especially those that shouldn’t exist.” People typically respond that they were not very good at chemistry at school—or they inquire about explosions and smells. And there, usually, the conversation ends.
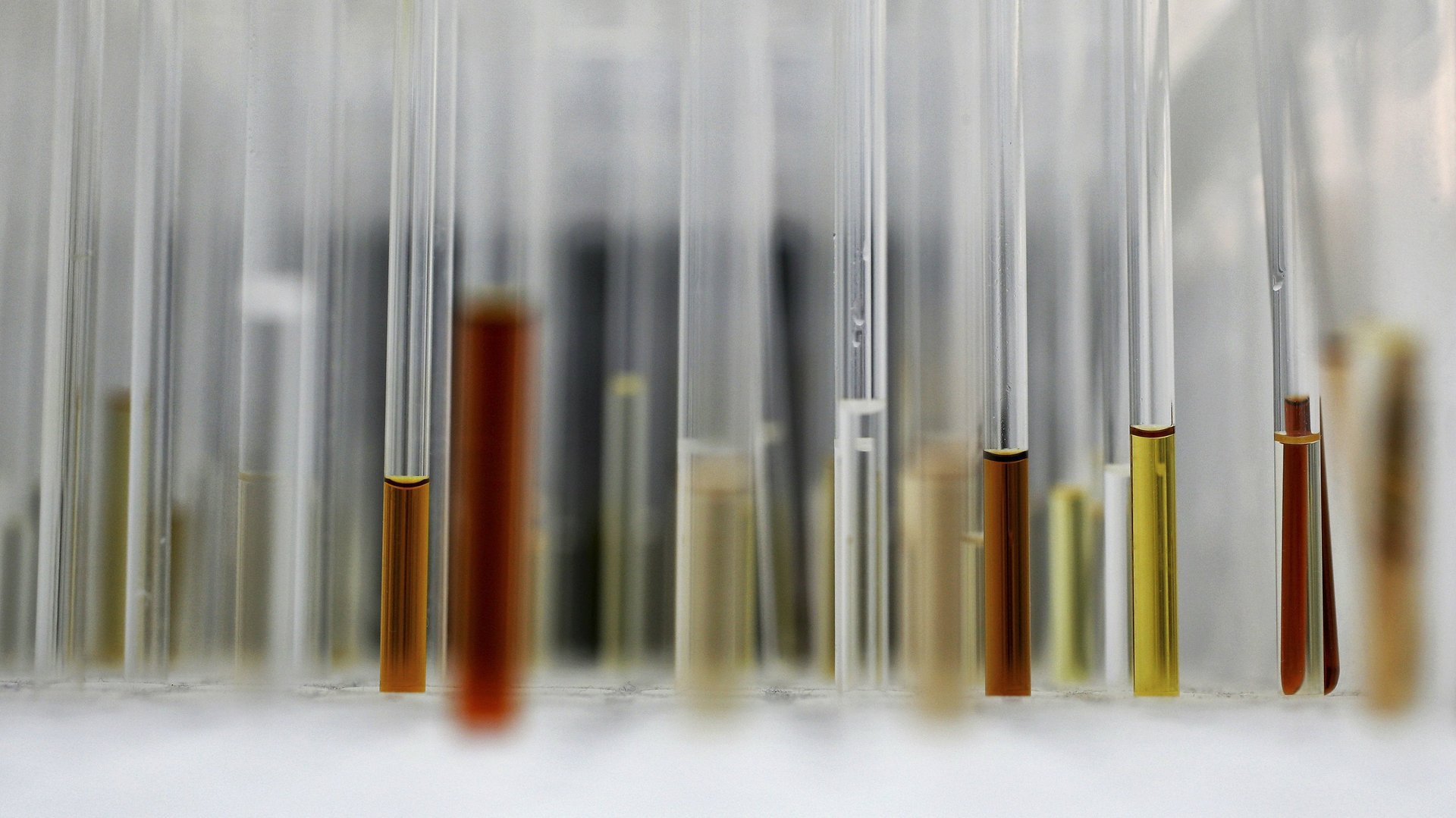
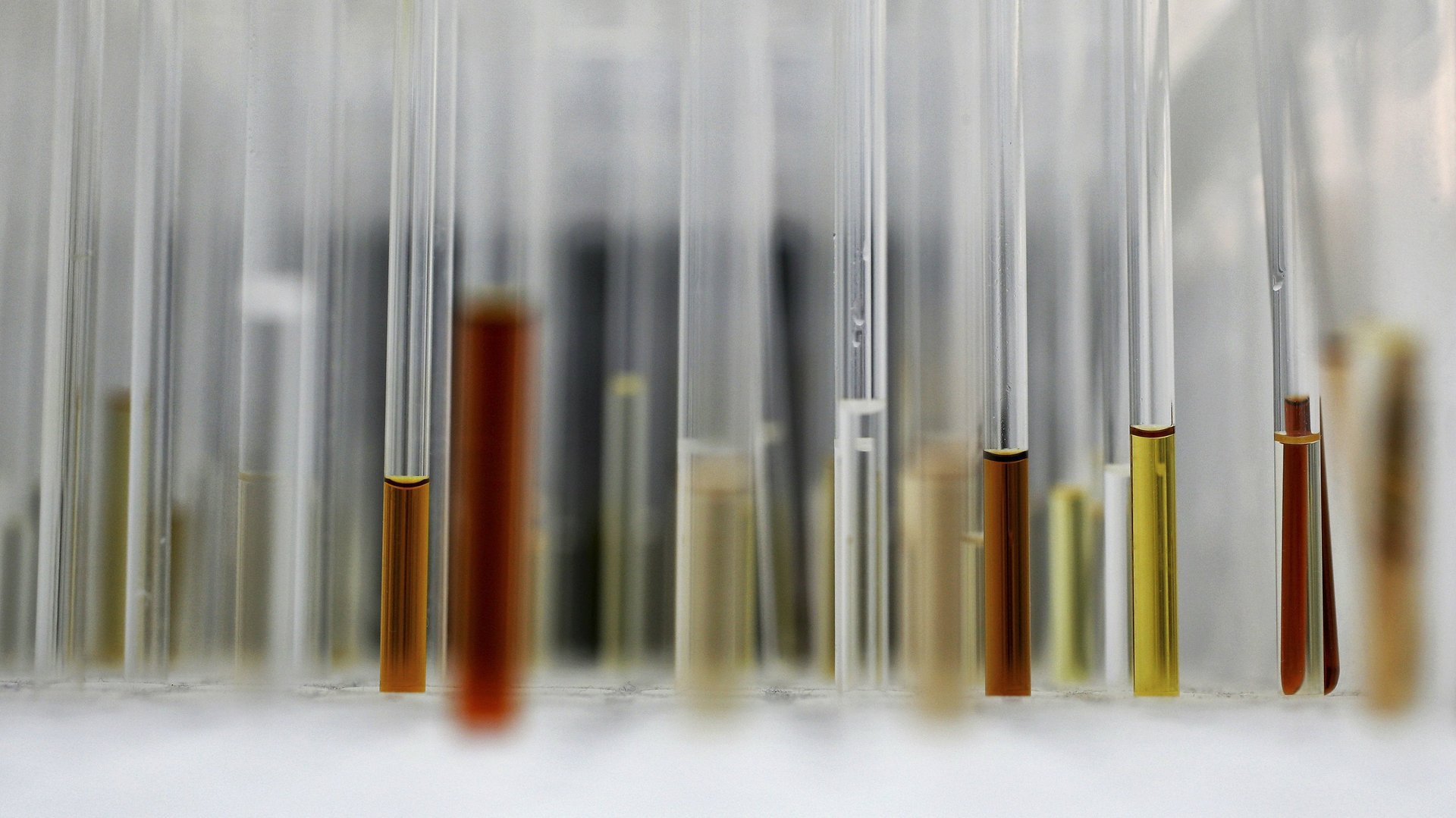
At drinks parties and dinners, if someone asks what I do for a living, I always say: “Synthetic chemist … I make new molecules … especially those that shouldn’t exist.” People typically respond that they were not very good at chemistry at school—or they inquire about explosions and smells. And there, usually, the conversation ends.
I worry that chemists are missing a self-promotion trick. While physicists can argue the need to understand the fundamental nature of the universe by studying subatomic particles at the Large Hadron Collider, we chemists beaver away using and developing fundamental knowledge of how to connect molecules together. We routinely have to overcome basic thermodynamics, which would stop any of us from existing if they controlled the universe—the building blocks of life would simply end up as carbon dioxide, water, and ammonia.
I suspect chemistry’s problem is that much of it is just too useful and everyday—though not all of it, as we shall see. Chemistry tends to have recognizable applications such as making drugs, paints, plastic, synthetic fibers, and electronics. The Hadron Collider, on the other hand, benefits from looking spectacular and performing abstract feats, the appeal of which lies in its distance from the world that we know.
My work
For the past 40 years, I have worked on the chemistry of the heavier group 16 elements, including sulphur, selenium, and tellurium. These have always fascinated me—in part, because the reaction chemistry is quite unpredictable. My early work was on sulphur-nitrogen compounds. Sulphur and nitrogen are quite unusual in that they both exist in nature as their basic elements. With some ingenuity, it is possible to form simple compounds containing only them—a classic case of overcoming the thermodynamics that are responsible for the elements being “stable.”
One example is tetrasulphur tetranitride (S4N4), an orange solid with an interesting cage structure that was first made 180 years ago. The compound is perfectly stable—at least, unless there is a tiny bit of heat from friction. That makes it explode violently into sulphur and nitrogen as thermodynamics takes over.
One of the main uses of tetrasulphur tetranitride is as a precursor to creating other sulphur-nitrogen compounds. It can be used, for instance, to prepare a longer chain-like molecule known as a polymer. This is truly alchemy. Known to chemists as polythiazyl, it looks metallic and golden and conducts electricity.
Polythiazyl was in fact the first non-metal material found to be a superconductor at low temperatures. Its discovery was partly responsible for the whole era of organic electronic materials, and apart from winning its discoverers a Nobel Prize, has given us technology like OLED televisions, which need no backlight and can therefore be thinner and lighter than other televisions. How ingenious was the synthetic chemist who took two stable, highly-abundant elements and prepared a simple compound that otherwise would not exist?
Phosphorus and tellurium
My group has also worked on phosphorus-sulphur chemistry, which is important to the additives that keep engine oil from turning into tar; and phosphorus-selenium chemistry, which is behind glass-like semiconductors—these might have applications in solar cells in the longer term. Most recently we have been trying to make simple compounds that bond together phosphorus and tellurium (known as P-Te bonds). This is a stupidly hard thing to do. The customary wisdom is that because tellurium is metallic, simple P-Te bonds are bound to simply break and leave you with tellurium metal. To demonstrate otherwise, it took three groups working together in Canada, Germany, and the UK.
I cannot envision any ready practical application for such compounds, but that certainly doesn’t make it pointless. Learning how to manipulate the structure to stabilize a P-Te bond gives us a fundamental understanding of the forces that hold molecules together and the reaction pathways that can be employed, which is transferable to other problems in chemistry such as making stable materials for the electronics industry.
Along the way we developed new routes to involve certain chemicals in the process. These may prove very valuable for others working in more applied areas (it’s hard to predict what these might be). We learned that an unlikely bond is perfectly possible and can be created in compounds that can be weighed out and put on the shelf. It was also a challenging project for the PhD student who did most of the work.
If you want to understand the art and science of chemistry, this sort of work sums it up; we’re making molecules that shouldn’t exist. It may not lay claim to answering life’s big questions in the same way as physics, but we are still talking about explorations in science that often benefit us in more ways than we can predict. When it comes down to it, the two disciplines are really not so different.
As for my team, now that we have shown what can be done with phosphorus and tellurium, we are wondering: where next? Arsenic-tellurium compounds are even more challenging, so watch this space.
This post originally appeared at The Conversation. Follow @ConversationUS on Twitter. We welcome your comments at [email protected].