Even if you never heard of Vera Rubin before her death on Christmas Day, you almost certainly heard of dark matter. Rubin was the astronomer who found it, and invented an entire field of study in the process.
In the late 1960s, Rubin had been working with fellow astronomer Kent Ford, taking spectrographs of distant objects, galaxies among them. Spectrographs separate the light of an object into a rainbow, and from that one can see the target’s motion via the Doppler effect. The Doppler effect is why a car engine or siren sounds like its pitch is higher when it approaches and lower when it passes. In those cases, the sound waves’ peaks are closer together as the car approaches and stretched out it goes by, resulting in higher-frequency sound followed by lower-frequency sound. The same thing happens to light, and it’s how we know that the universe is expanding: the light of galaxies and quasars in the distant parts of space are shifted to the red, lower-frequency part of the spectrum.
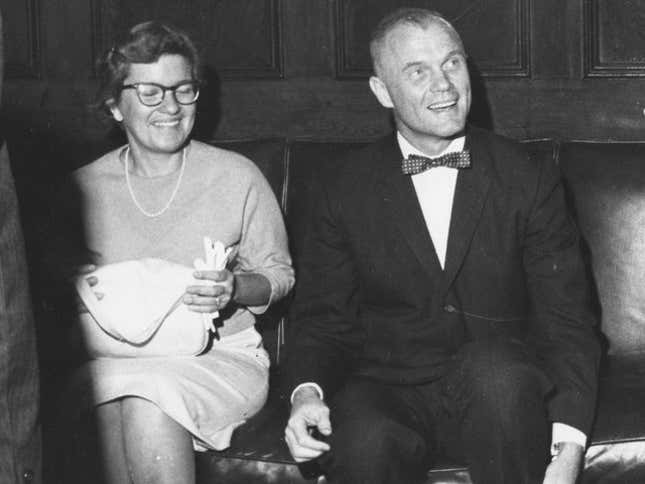
But there’s another thing the spectra can tell you: if you zoom in on a galaxy closely enough, you can see the Doppler shift in different parts of it. The parts moving towards the observer are bluer than normal, while the parts moving away are redder, and that tells you how fast the galaxy (or at least the visible stuff in it) is rotating. Rubin trained her spectrograph on Andromeda, the closest large galaxy to us.
Rubin found that certain bright regions of the galaxy seemed to all be revolving around its center at the same speed. Newton’s law of gravity says that if you’re in orbit around a heavier mass in space, you should move more slowly the further away that mass is. In the solar system that’s exactly what happens: Pluto takes 248 Earth-years to go around the Sun, while Mercury (not that much bigger than Pluto) takes just 88 Earth-days, since it’s so close to the Sun. Velocity measurements plotted against distance from the center of a solar system, or galaxy, are called a rotation curve, and it should slope gently downward. But Andromeda’s was flat.
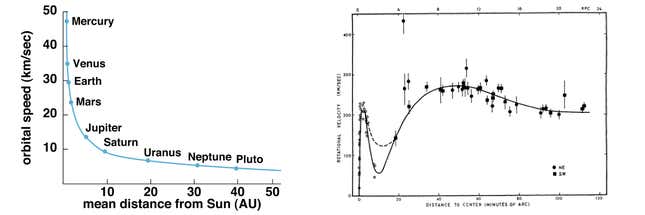
To be sure this wasn’t a fluke Rubin and Ford observed dozens of other galaxies. And the data were clear: the rotation curves for galaxies were flat. Another anomaly was how much stuff was in the galaxies themselves. The luminous matter astronomers could see wasn’t enough to hold the stars in place, especially if the rotation curves Rubin measured were correct.
That’s a big reason why Rubin’s work is so influential in astronomy, says Risa Wechsler, an associate professor of physics at Stanford who studies models of dark matter, what it might be and how it affects the evolution of the cosmos as a whole. “It’s one of the most important discoveries of the 20th century,” says Wechsler. “She basically showed that most of the mass in the universe is not emitting light.”
Rubin wasn’t the first person to notice anomalies, but she did some of the first large-scale studies that showed the phenomenon wasn’t just an observational error that would disappear eventually. Coupled with independent observations from Albert Bosma in the Netherlands, who studied cold gases rotating at the outer edges of galaxies, and additional theoretical work in the mid-70s, the result was a whole new field of study.
“It gets into the question of why we are here,” Wechsler says. Absent dark matter, the gases in an expanding universe would never coalesce to form anything denser—the cosmos would be full of diffuse gas. “You essentially need that extra gravity that dark matter provides to get the gas in the universe to collapse and form stars ” No stars, no life.
Her observation was fundamental, yet Rubin never got a Nobel Prize. There could be a number of factors at work. One is simply sexism: there have been only two women awarded a Nobel in physics in the history of the award, Marie Curie in 1903 and Maria Goeppert-Mayer in 1963. That’s two out of 203 winners in the field. And a prominent case of a Nobel “snub” has been Jocelyn Bell, a female astrophysicist who was instrumental in discovering pulsars, rapidly rotating cores of dead stars. Her thesis advisor Antony Hewish won the award for that exact finding in 1974 (along with astronomer Martin Ryle), even though Burnell was the one who had pored over radio telescope data to make the initial discovery.
During her lifetime Rubin had to deal with casual sexism in a number of areas; she told physicist Alan Lightman that early in her career, a male department chairman offered to present one of her papers at a conference —in his name. Rubin said no.
Another is a bias against astronomy. Wechsler notes that there initially wasn’t really much consideration for the field in the first decades of the Nobel, though that has changed somewhat. Hans Bethe got a Nobel in 1967 for his work on nuclear reactions that power stars, and since then six more Nobels in physics have gone for astronomy-related work. The last was in 2011 when Adam Riess, Brian Schmidt, and Saul Perlmutter got the nod for their discovery that the universal expansion is accelerating.