For half a century, neuroscientists thought they knew how memory worked. They were wrong
We rely on memory to pass exams and excel at trivia nights, but in fact the stakes are far higher than simply recalling facts, no matter how essential. Memory structures our identity; it’s the foundation of consciousness. Our past and current selves, emotions, and experiences, are linked together thanks to memory.
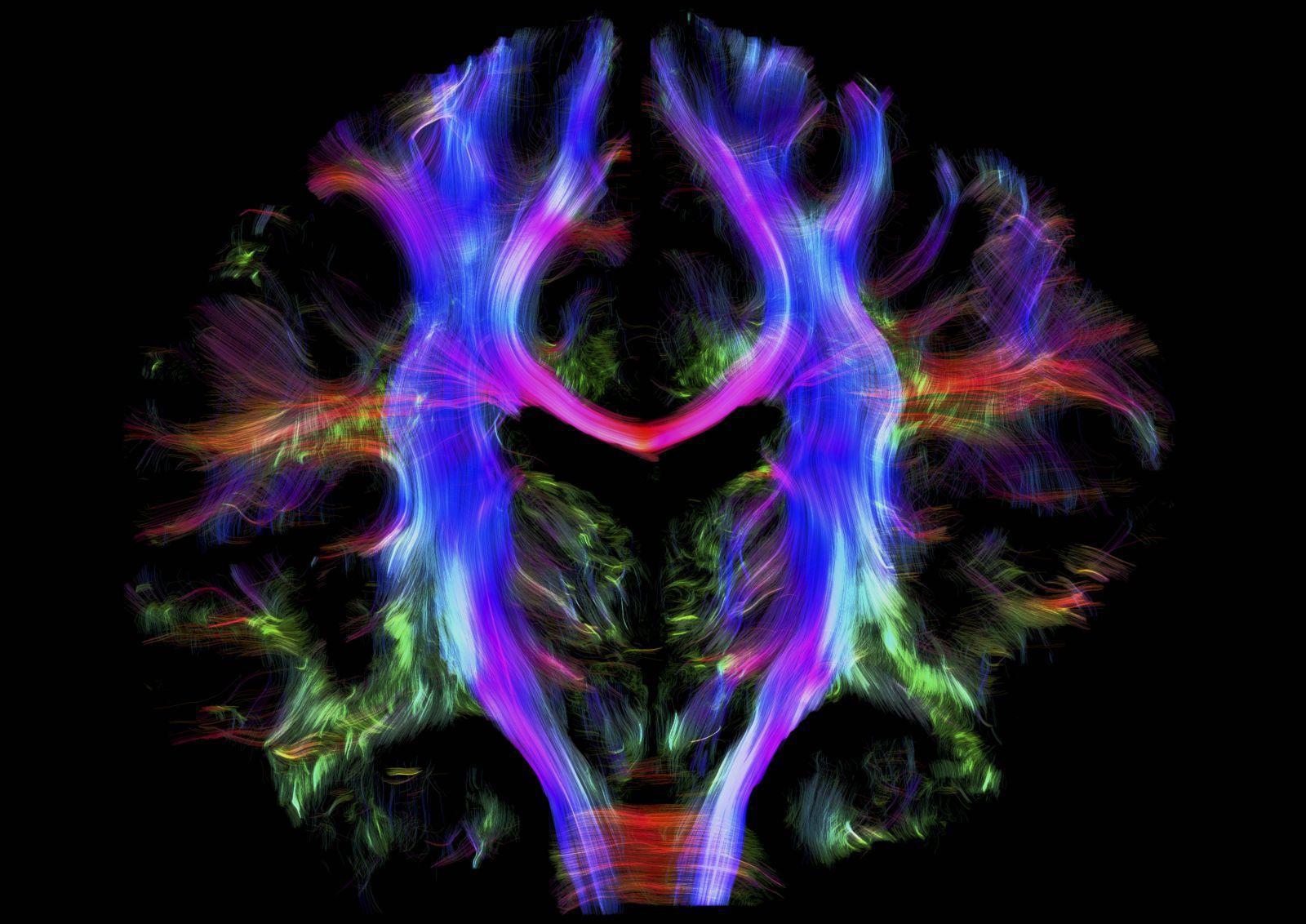
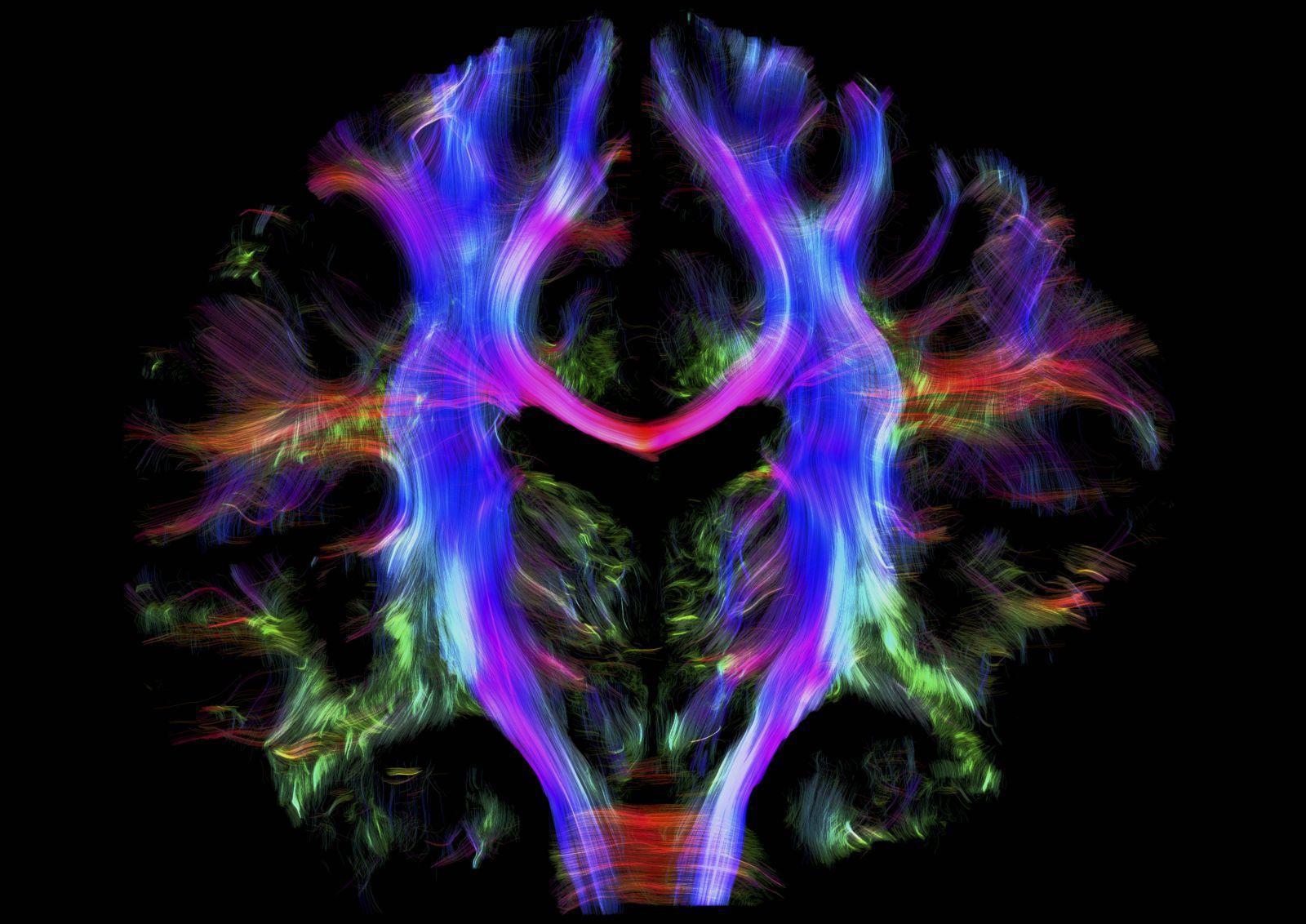
We rely on memory to pass exams and excel at trivia nights, but in fact the stakes are far higher than simply recalling facts, no matter how essential. Memory structures our identity; it’s the foundation of consciousness. Our past and current selves, emotions, and experiences, are linked together thanks to memory.
Which is why a study that rewrites scientists’ understanding of how memory works is so intriguing and potentially transformative.
It’s long been known that different areas of the brain have different functions when it comes to storing memories. The hippocampus stores short-term memories while the prefrontal cortex stores long-term memories. Scientists have known this since 1953, when a lobotomy attempting to cure epilepsy — involving the removal of significant portions of the hippocampus— left patient Henry Molaison unable to remember new experiences, but with perfect long-term memory.
But for decades, neuroscientists believed that short-term memories were transferred from the hippocampus to the prefrontal cortex, where they became long-term memories, slowly over time. Now, a study by scientists at the Riken-MIT Center for Neural Circuit Genetics in Tokyo provides strong evidence that short- and long-term memories are formed in the brain simultaneously.
The study, published last week in the journal Science, was conducted on mice, though all mammalian brains have a similar memory structure and the scientists expect the findings to apply to humans.
In 2012, Riken-MIT researchers were able to identify the neurons involved in storing specific memories, which they called “engram” cells (an “engram” is used in scientific literature to describe the physical existence of a memory stored in the brain.) Once they identified these engram cells, the researchers were then able to map out the circuits activated when remembering certain events. In the most recent study, scientists used optogenetics to artificially turn off and on particular neurons, and so see when memories in each area of the brain became active.
The researchers, led by Takashi Kitamura, created a memory in mice by giving them an electric shock. One day after the event, the mice had identifiable memory circuits about the event in both the hippocampus and the prefrontal cortex. But, crucially, if the short-term memory in the hippocampus was turned off, the mice couldn’t remember the shock at first. The long-term prefrontal cortex memory was initially “silent” and, only when the scientists artificially activated it using optogenetics, could the mice remember the shock using the long-term memory. That, the researchers say, shows that the long-term memory is created at the same time as the short-term memory—even if the long-term version is silent at first.
Kitamura believes it makes sense for the two memories to be formed simultaneously as they each have a different but hugely important function. Short-term memory is certainly needed: “When an animal experiences an episode, the animal’s brain encodes detail and vivid memory for this episode,” Kitamura writes in an email. But the long-term memory has a different role: “The brain also encodes the rule memory or relevancy for this episode (e.g. BoxA = danger),” he explains.
Humans don’t typically go around experiencing electric shocks, but Axel Cleeremans, cognitive psychologist at Université Libre de Bruxelles who was not involved in the study, has a more everyday explanation: Parking a car.
Short-term memory, Cleeremans says, allows you to find the specific street and space where you parked the car that day. But long-term memory accumulates all these individual instances into a broad set of data, so you know the streets where you’re most likely to find a parking space and enables you to develop a great car-parking strategy.
The findings could be crucial for treating memory-related illnesses. “In this study, we found that memory engram cells naturally change their status from active to silent ([in the] hippocampus) and from silent to active ([in the] cortex),” says Kitamura. “If we identify neural mechanisms how silent memory can be active, we may find a treatment for amnesia/forgetting.” Conversely, the same knowledge could be used to help silence especially debilitating traumatic memories in PTSD patients.
Potentially, these findings could shape how we view the working of the brain overall. Cleeremans believes the study supports “higher-order thought” theory of consciousness, an assessment Takashi agrees with. The theory posits that we become conscious of experiences and states when a higher level of mental state has representations of it. So, for example, if you lose a watch your grandfather gave you, you likely will feel awful about it. But then as you go about your day, week, and month, you’ll likely forget the pain of that loss—though, at times, something will trigger you to become conscious again of that experience, and you’ll feel bad again. The consciousness of the loss of the watch is the higher-order thought; the feeling of loss itself is the lower-order state. Cleeremans explains that higher-order thought theory “assumes that patterns of neural activity in the posterior brain only become conscious when they are targeted by prefrontal neurons,” and this idea is supported by the Riken-MIT study’s findings on how long-term memories are activated.
This sounds complicated, and it is, but it’s also very cool. Cleeremans quotes neuroscientist Christof Koch as saying, “the front [of the brain] is looking at the back.” It’s a mind-bending concept (quite literally.) But unpacking the mechanics of memory can help us understand how our own minds work.